We are currently witnessing a dramatic decrease in biodiversity. Species virtually go extinct on a daily basis, some of them as yet undescribed and hence unnoticed. In fact, human impact on global biodiversity, as well as on the earth's climate and geochemistry, is so severe that a new geological epoch, the Anthropocene, seems to be justified.
However, this isn't the first time in the history of this planet that a severe loss of biodiversity has occurred. Earth has already witnessed five such mass extinctions in the distant past, all characterised by a staggering loss of at least 70% of all species. Nature has, however, always recovered with new species replacing the old forgotten ones, taking up the vacant ecological niches and often evolving strikingly convergent forms and functions. Evolution is a continuing process that has sometimes been accelerated by mass extinctions. As Charles Darwin stated so elegantly at the end of his book, “whilst this planet has gone cycling on according to the fixed law of gravity, from so simple a beginning endless forms most beautiful and most wonderful have been, and are being, evolved.” (On the Origin of Species, 1859, pp. 489-490).
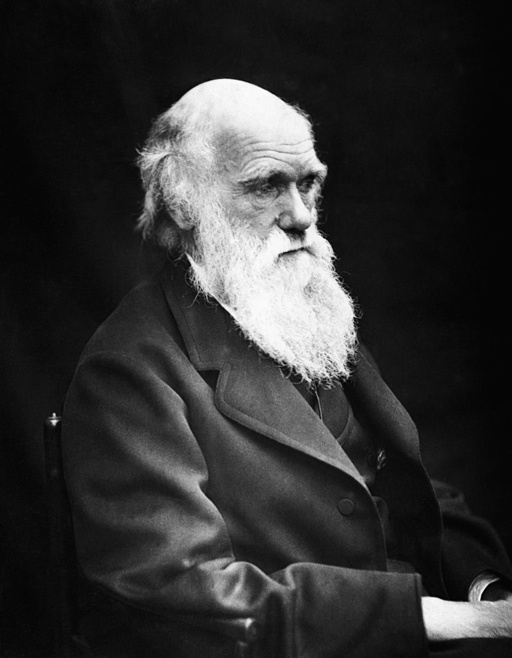
Why then should we worry about the ongoing extinctions and loss of natural landscapes? Climate change, pollution, and sea level rises together with over-harvesting of both oceans and land are certainly not worse than the events that caused the previous mass extinctions. These included the impact of a celestial body 10-15 km across (the Chicxulub impactor) and the volcanic eruption of flood basalts covering some 7,000,000 km2 (the Siberian Traps)! As was the case following previous mass extinctions, new life forms will also fill the remaining gaps this time, so why should we be alarmed? Why spend millions on endangered species while we can have new ones for free?
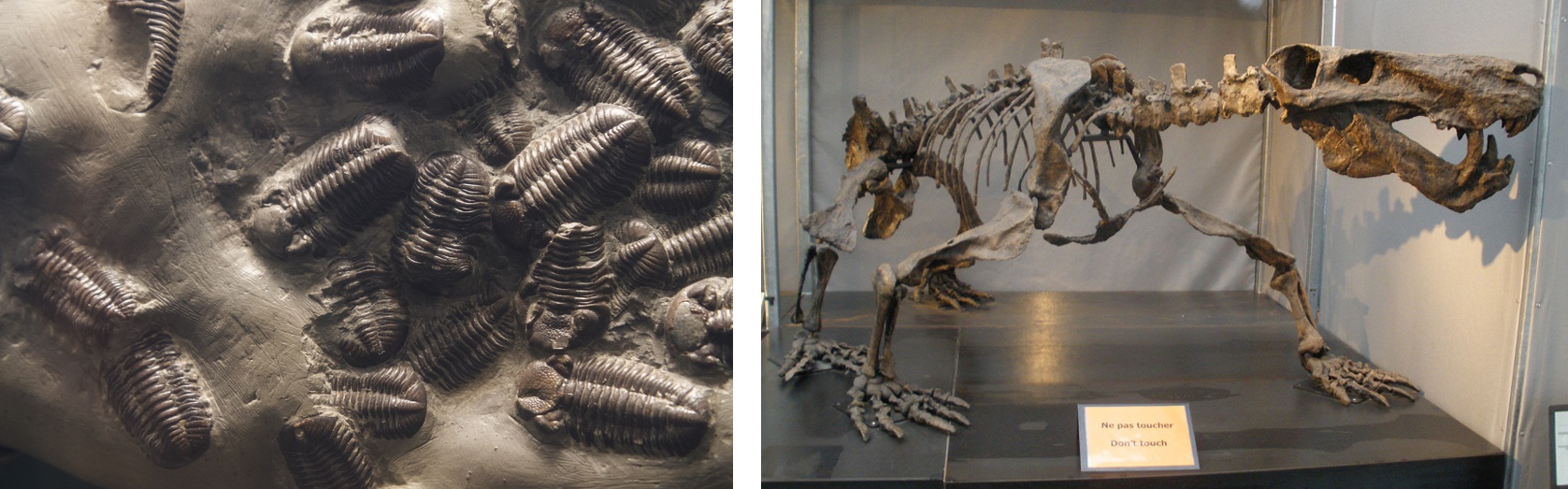
Unfortunately, this time around it is not quite so simple. Three issues come to mind. Firstly, time: Is there really enough time for the predicted massive turnover of biodiversity to occur? Secondly, what about the replacement of lost species by feral or other introduced species – can this really compensate for the loss of native species? Thirdly, as new (sub)species have already started to evolve in man-made ecosystems – is this likely to be a major mechanism for continuing speciation processes on a global scale? The answer to each of these questions is no!
Time is a most important prerequisite for new species to evolve. For example, it can take as long as 30 million years for an atoll to form. It can take 10,000 years for a reef to completely encircle an island and perhaps 100,000 years for a complete barrier reef to form. Any barrier reefs lost today will not be replaced within one or two generations, but will rather require of the order of 25,000 generations, which is way beyond our imagination. Such a huge delay between extinction and the evolution of new species is painfully demonstrated in Earth's fossil past. The previous five mass extinctions were all followed by an impressive radiation of new species, sometimes even referred to as “explosions” because of their range and the geologically short time spans required. Each period following such extinction events witnessed the rise of a new group of major life-forms on Earth, and was often named accordingly (e.g. the Age of Dinosaurs and the Age of Mammals). New species entered the spaces vacated by those that became extinct. Time, however, was always an important factor. After each extinction it took millions of years before biodiversity was restored to the pre-extinction level. Not the ten, or at most one hundred, years most people have in mind when talking about new species that they imagine would fill the vacancies left by the ongoing extinction of species. For example, some 10,000,000 years passed after the Great Dying (the Permian/Triassic extinction event) before the vacated ecological niches were again fully occupied. We don’t really have that much time to play with.
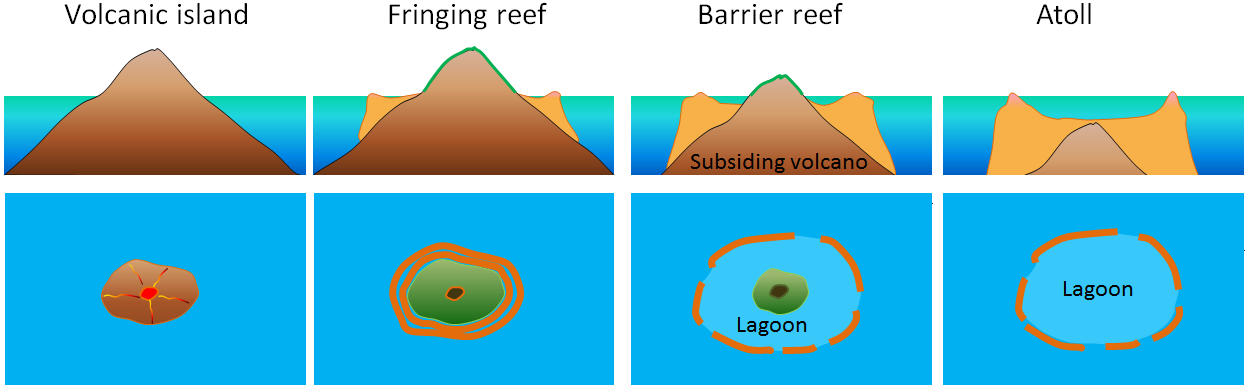
Actually, it’s even worse than that. The time required for recovery doesn’t start immediately after extinction events. Speciation is unlikely to occur while habitat fragmentation and degradation is still continuing: A new equilibrium has first to be achieved. This is best illustrated by species richness patterns on oceanic islands: During the early stages of an island’s life cycle, species richness increases because there are still a lot of vacant niches available (i.e. the island's carrying capacity exceeds its species richness). In contrast, during an island's final stages such as when it is in decline due to erosional attrition or subsidence, species richness decreases hand in hand with reductions in carrying capacity. Habitats on the continents are today declining and fragmenting in a similar way to that seen during the decline of oceanic islands, but due to the activities of our species. As long as this process continues the carrying capacity will only decrease, and so will be the total number of species. Even if new species (or more likely, subspecies or varieties) arise this is likely to coincide with the extinction of others.
Newcomers do, however, exist amidst the ongoing extinction events. So what are the potential mechanisms by which humans promote the formation of new species?
Relocation
New arrivals in deteriorating habitats are often introduced species, greatly assisted by humans in their global dispersal which would otherwise not have been possible. We also generously give them a good head start by providing them with additional shelter and food in our settlements and parks. Introduced species, a number of them invasive, are flourishing everywhere and taking over the ecological roles of native species that either have already disappeared or are still present but in decline. For example, feral red deer (Cervus elaphus corsicanus) introduced into Sardinia and Corsica now roam the forests where, until about 10,000 years ago, the native dwarf deer (Praemegaceros cazioti) lived, herds of feral horses and camels form an integral part of Australia’s wilderness replacing various species of long-gone giant marsupials including the giant wombat (Diprotodon optatum) and the giant short-faced kangaroo (Procoptodon goliah), and fallow deer (Dama dama) are now browsing New Zealand forests instead of the large, extinct, flightless birds called moas (nine species of Dinornithiformes).
One herbivore or the other, does it really matter? Some ecologists claim that the number of species per area remains the same or has even increased. New Zealand now has more mammals than ever (it used to have none, except for three endemic bat species of which one is believed to have recently become extinct) and birds were the only warm-blooded vertebrates until our species arrived at about 1,300 CE. But species numbers are misleading in this case. They don’t capture the fact that the very same species are often introduced in many different areas, replacing the native species that were unique to their specific locations. On a planetary scale this translates into a dramatic reduction in biodiversity. Wherever we go we meet the familiar suite of species; feral goats, red and fallow deer, rabbits, commensal rats and mice, sparrows, mallards, and many more. At the same time the landscapes also begin to look more and more similar, with rural grasslands and meadows, secondary or tertiary forests, artificial lakes, and canalized waterways. In short, the earth is losing unique landscapes, including its native flora and fauna, only to be replaced by controllable habitats and species. We and our domesticated animals constitute 96% of the total mammalian biomass on Earth . That not only sounds rather boring, it’s also risky. One fatal epidemic and an entire species can vanish.
Over sufficiently long time periods introduced species may well develop into novel (sub)species that are distinct from their parental founder populations, as has occurred on Mediterranean islands with the mouflons that descend from domesticated sheep, and in Australia with domesticated dogs that turned feral and evolved into present-day dingoes, but such speciation processes typically take several thousand years, also depending on generation times.
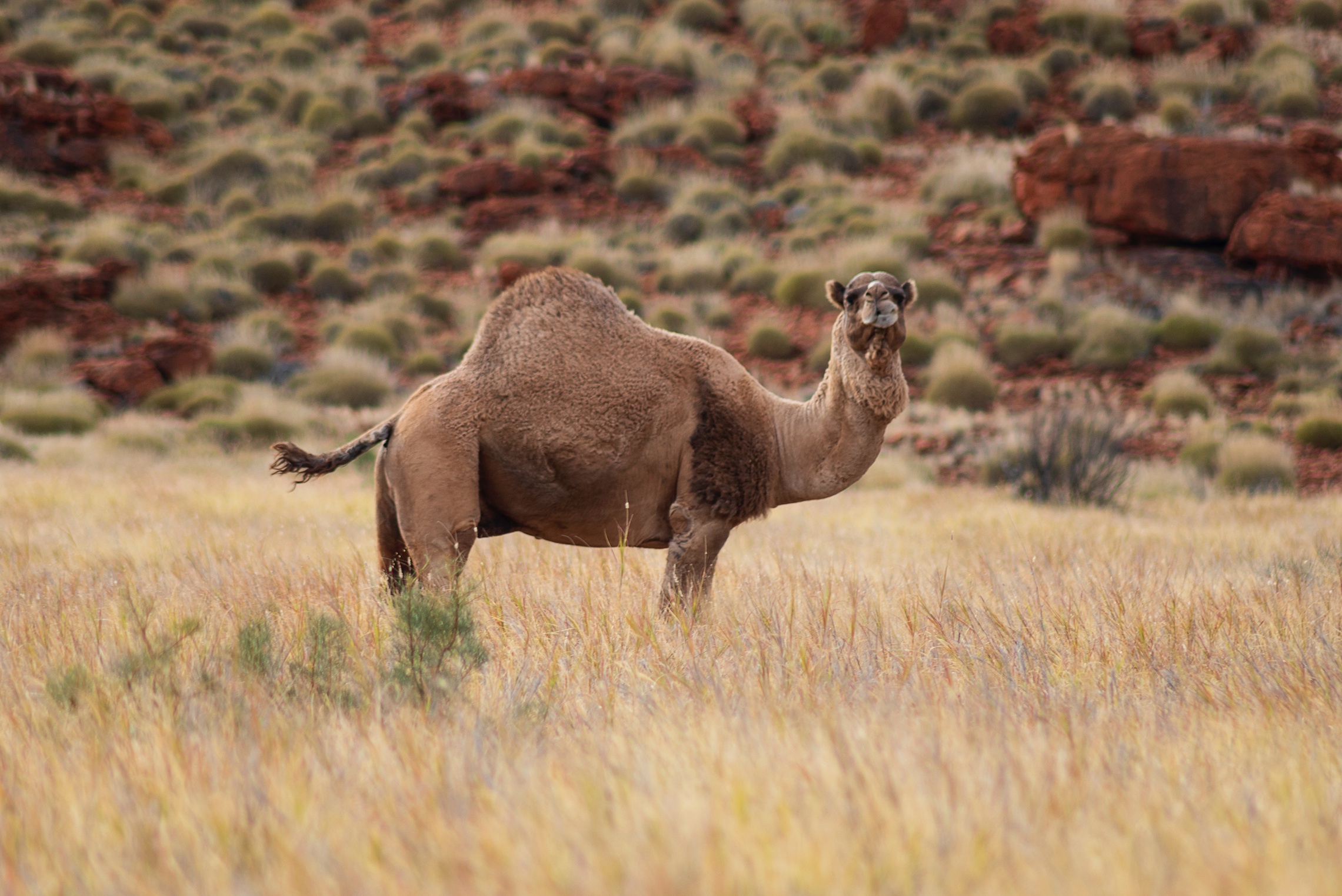
Novel man-made ecosystems
Humans not only destroy ecosystems, they also create new ones suited to their own particular needs. Prime examples of such novel man-made ecosystems can be found in urban landscapes. Species residing in adjacent territories sometimes move into such novel ecological niches and begin to adapt to their new environments. House finches (Haemorhous mexicanus), for instance, originally resided only in Mexico but have now spread over most of urban and suburban North America, adjusting to this ecosystem by developing longer beaks and by singing at a lower frequency in order to overcome background city noise. Similar adaptations have been reported from great tits (Parus major), which have also developed longer beaks, together with the habit of feeding from garden bird feeders. Urban crested anoles (Anolis cristatellus) developed longer limbs and a better grip for climbing on city buildings, while common starlings (Sturnus vulgaris) developed rounder wings that enabled them to take off more quickly in order to escape cars and cats.
It's not simply a matter of documenting morphological and behavioral adaptations in species that move from rural environments into urban environments; evolutionary biologists have also started to pinpoint the underlying genetic changes. A good example is the common blackbird (Turdus merula): populations of blackbirds across Europe have increasingly favored city life over rural life, becoming more daring, less migratory, and breeding earlier in the year. Blackbirds are at present one of the most common urban bird species in Europe, but 150 years ago they lived mainly in forests. A study that compared the genetic makeup of urban blackbirds with that of forest-dwelling conspecifics found differences in the genes of urban populations that are implicated in brain biochemistry and associated with harm avoidance behavior (i.e. flight initiation distance) . The urban gene variants also occur in the original rural blackbird population but with a much lower frequency, indicating that the new urban environment selects for a brain biochemistry that reduces the flight initiation distance, thereby reducing the time and energy wasted when constantly having to flee approaching cars or people.
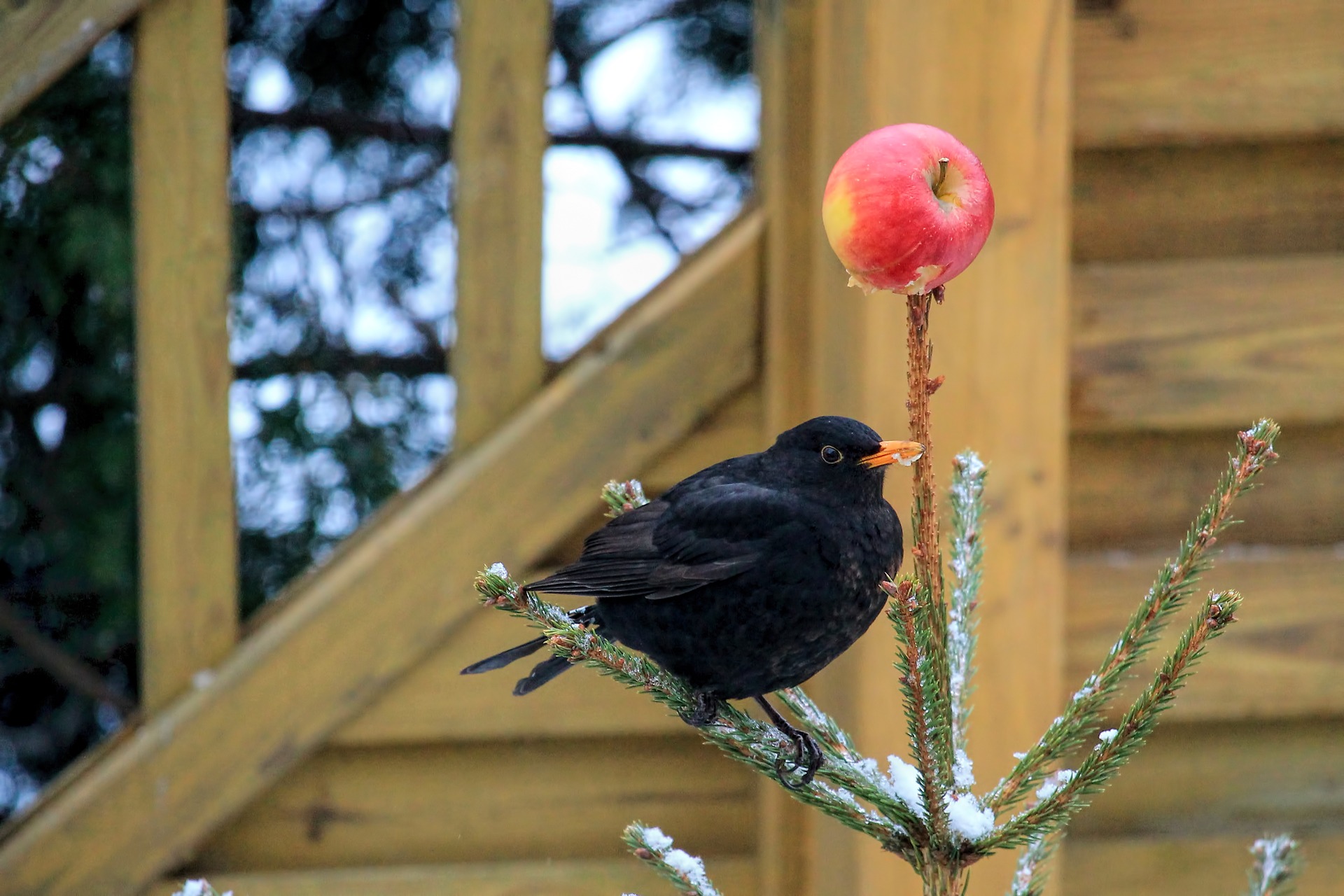
In New York City the white-footed mouse (Peromyscus leucopus) has formed a local population that is genetically adapted to fat-rich fast foods as its nutritional source . While these adaptations to urban life have not yet led to genuinely new species, this is exactly what seems to have happened in the London tube. The common house mosquito (Culex pipiens) has adapted to the environment of the underground railway system and sewers, establishing a subterranean population. Now named the "London Underground mosquito", Culex pipiens molestus can no longer interbreed with its above-ground counterpart and, by definition, should therefore be regarded a species of its own . An alternative interpretation, however, is that the underground mosquito, which is not limited to London but is found in underground systems worldwide, is in actual fact a southern mosquito (Culex molestus) that has spread to northern latitudes taking advantage of these relatively warm locations, where winter is unknown and food – in the form of rats, mice and humans – is plentiful .
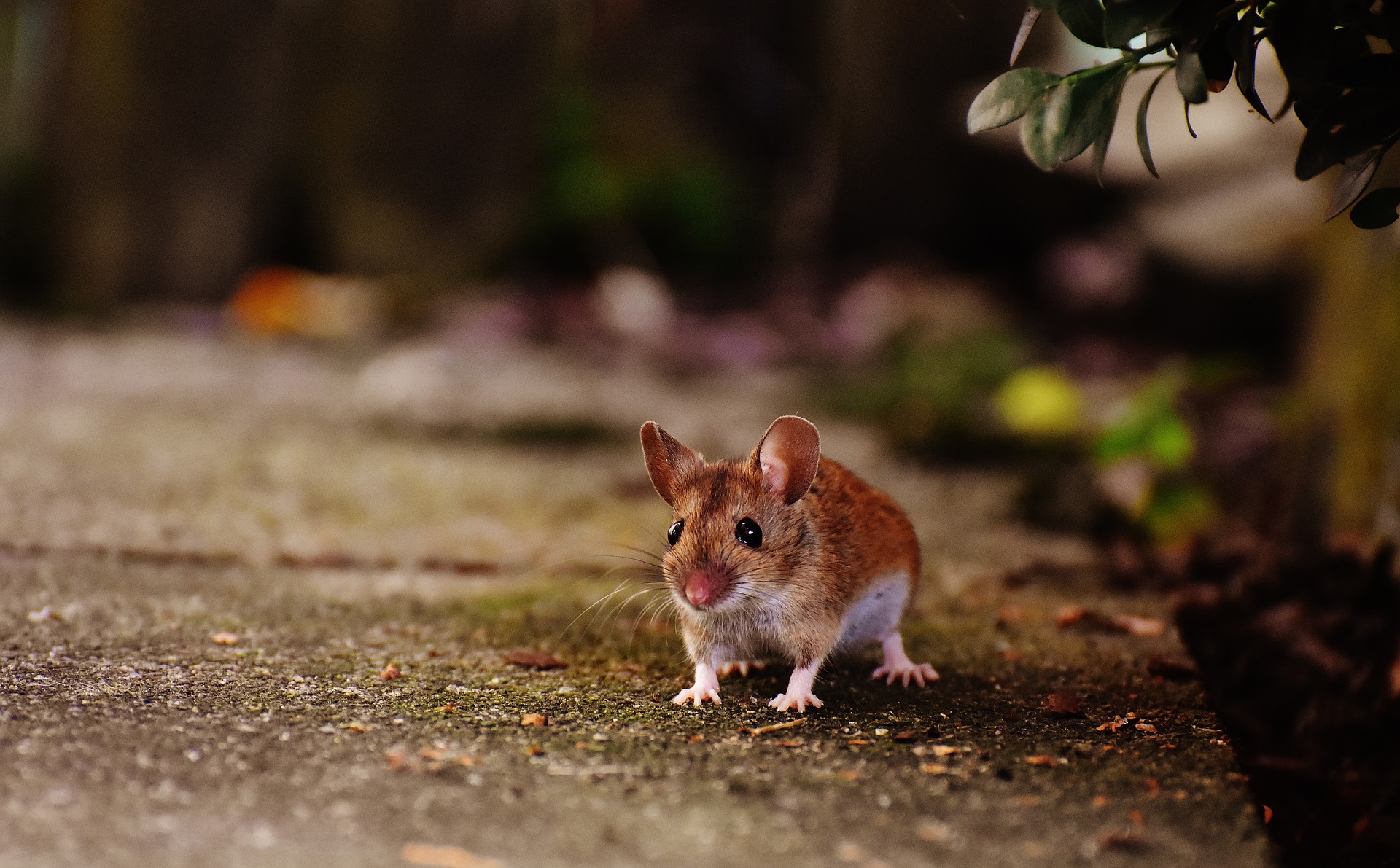
It is important to note that these examples are rare cases compared to global biodiversity levels. As indicated above, these new varieties in most cases cannot be considered to represent new subspecies, let alone new species, but in times to come they may well form genuine new species and thus replace some of today’s lost biodiversity.
Taken together, these tiny evolutionary steps suggest that cities may be potent drivers of adaptive evolution. Sometimes these new forms result by selection of mutations that were already present, albeit at low frequencies, before urbanization occurred (as is the case for common blackbirds, Turdus merula). In other cases new mutations may have been responsible, as with the peppered moth, Biston betularia.
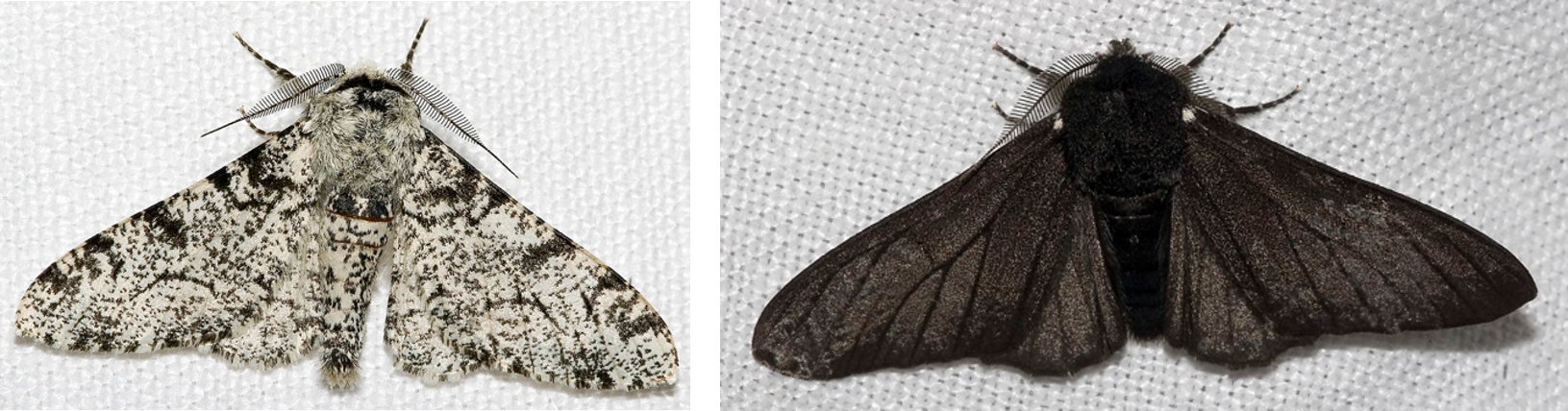
Selective hunting
Non-adaptive evolution, often a result of random genetic drift and/or restricted gene flow, can also be man-made. This frequently leads to increased genetic differences between populations, but the drawback is that the new population typically suffers from a loss of genetic diversity. Take for example the world's largest wild herd of white-tailed deer at the former Seneca Army Depot in New York State. These are leucistic forms (not albinos; the eyes are black) of the common, wide-spread, white-tailed deer (Odocoileus virginianus). This population is the result of a set of recessive genes that were already present in the ancestral population. The lack of predators combined with selective hunting of the brown individuals resulted in an increasing proportion of individuals carrying those particular genes. Given sufficient time, such isolated forms certainly could have the potential to gradually deviate more and more from the founder population and to eventually form a new species in its own right – in this case deer that are all-white and not just white-tailed. But should we aspire this? The aberrant colour on its own is not the biggest problem, even though it is unnatural. The problem lies in the reduced genetic variation. This may lead to inbreeding depression, reduced fitness, reduced ability to adapt to novel environments, and once again, to extinction.
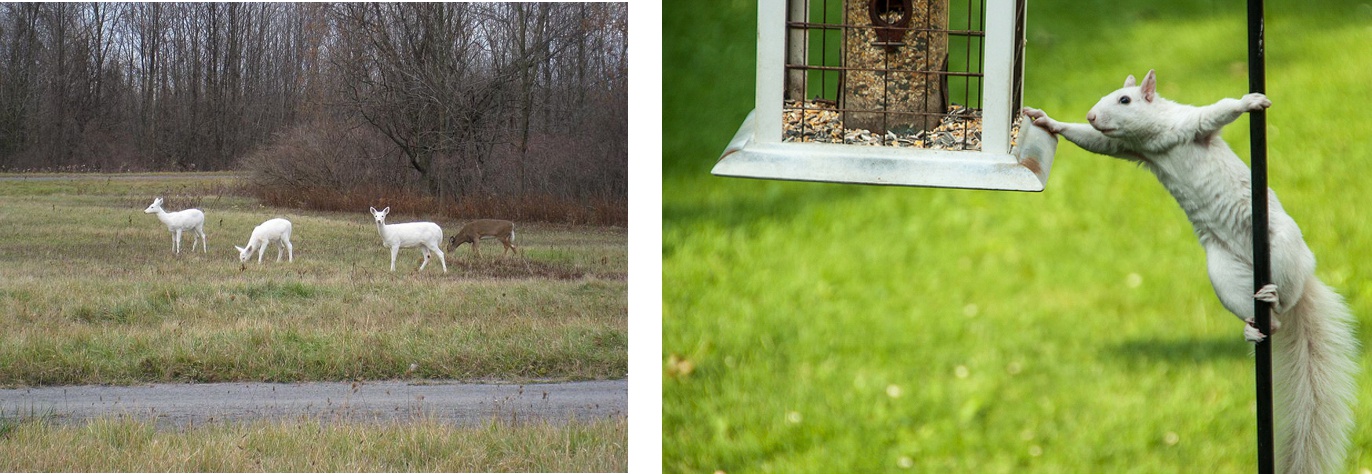
The story of life on Earth is ever continuing with its ups and downs, some massive and some minute, but if we carry on the way that we are currently we will not witness the next "up" part for millions of generations to come.
Further reading on this topic:
- Schilthuizen M (2018) Darwin Comes to Town: How the Urban Jungle Drives Evolution Picador
- Thomas CD (2017) Inheritors of the Earth: How Nature Is Thriving in an Age of Extinction Penguin Books
- Johnson MTJ and Munshi-South J (2017) Evolution of life in urban environments Science 358: eaam8327
Responses