Characterizing Exoplanets: Where Are We and What Will Come Next?
By Franck Marchis in
Twenty-two years ago, after completing a few nights of observations at the Observatoire de Haute-Provence in France, Swiss astronomers Michel Mayor and Didier Queloz reported the strange periodic wobbling of the sun-like star 51 Peg . They concluded that this signal was caused by a massive Jupiter-like extra-solar planet (or exoplanet) in orbit around a fairly anonymous star 57 light-years away from us that was barely visible to the naked eye.
This discovery, made by a technique called radial velocity, was controversial for several years, but for two reasons soon became a game changer in the field of astronomy. First, 51 Peg b was the first confirmed exoplanet discovered around a star like ours. Previous exoplanets had been detected around pulsars, the remnants of dead stars. 51 Peg, the star of the planetary system, is an almost twin of our sun, 10% more massive and 1.5 billion years older. The existence of 51 Peg b confirms that planets around stars like our sun do exist and, to recognize this discovery, in 2015 the planet was officially named Dimidium. Secondly, Dimidium travels in a very tight orbit of less than four days, at only 8 million kilometers from the star - seven times closer than Mercury is to ours. Using models based on observations of Jupiter, theorists used to think that gas giant planets form far away from their stars and remain there. But the first exoplanet ever discovered fundamentally challenged our understanding of the formation and evolution of exoplanets. Today we know many “Hot Jupiters” like Dimidium, and assume that they migrated closer to their star a few million years after their formation.
A floodgate of discoveries
After this event, a floodgate of exoplanet discoveries burst open. Astronomers and talented engineers designed instruments able to detect the faint dimming of light due to the passage of an exoplanet in front of its star. This so-called transit method enjoyed incredible success in space starting in 2009, after NASA launched Kepler . The space telescope has been designed for one simple task, to stare at a field twenty times the size of the moon and monitor the brightness of about 150,000 stars, 80% of them similar to our sun, for four years.
The number of exoplanets detected since Kepler’s launch increases almost daily and to keep up with those discoveries the NASA Exoplanet Archive provides an updated list of known exoplanets and their characteristics, along with a set of pre-generated plots for astronomers and the public. The numbers are astonishing for such a young field - today we know of 3,472 confirmed exoplanets (as of April 21st 2017), 2,331 of which were discovered by the Kepler mission, and a thousand more should be soon confirmed. But what do we really know about those exoplanets?
Earlier, I described two main techniques used by astronomers to search and characterize exoplanets. Radial velocity, which brought to us 630 exoplanets, provides information on the orbit of the planets (their period, eccentricity, and distance to its sun) as well as a constraint to their mass. Because of its bias toward massive bodies, this method has detected 276 exoplanets similar to Jupiter in mass or higher.
The transit method has discovered 2,742 exoplanets, and permits us to know not just the measurement of the planet's period and distance to its host star but also its size. The NASA Kepler mission has provided a detailed census of exoplanets in our galaxy, with a total sample of 4,582 candidates and confirmed exoplanets, including 21% Earth-sized (R ≤ 1.25 x REarth) planets, 29% Super-Earths (1.25 x REarth < R ≤ 2 x REarth), 40% icy giants like Neptune/Uranus (2 x REarth < R ≤ 6 x REarth) and finally 10% gas giant exoplanets (like Jupiter).
Using another technique, gravitational microlensing, which consists of detecting the amplification of brightness of a star when it is occulted by another one and/or by a bounded exoplanet that acts as a gravitational lens, has led to the detection of forty-four exoplanets. Even though this number is relatively small compared to the ones described above, microlensing gives us an unbiased way to directly estimate the number of exoplanets per star. Today we know that, on average, there is
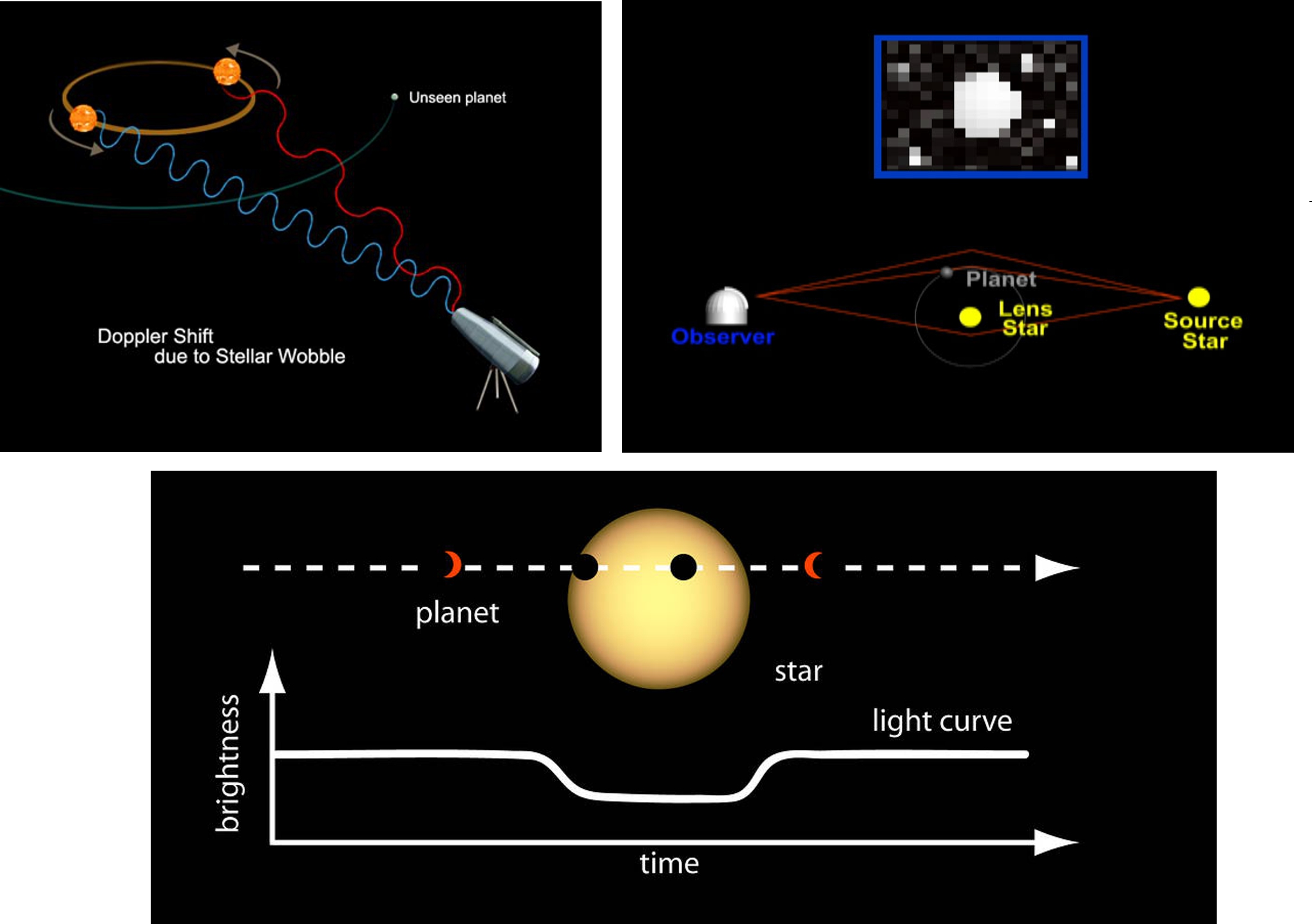
at least one planet per star . Combining this estimate with Kepler’s statistical survey, astronomers estimate that 20% of sun-like stars have a planet similar in size to Earth located in the habitable zone of its star . More impressively, recent studies claim that one in four M dwarf stars , the most common stars in our galaxy, host a small and temperate exoplanet. That’s a lot of potentially-habitable worlds in our galaxy.
The complex problem of habitability
An exoplanet is called potentially-habitable if it is located in the Goldilocks zone of its star , where liquid water could exist if the exoplanet has a decent atmosphere. But “habitable” does not mean, “inhabited.” Let’s have a look at our own solar system for example. Mars is habitable, and recent observations of its surface using rovers and images from orbiters suggest that the Red Planet probably had a large ocean and a dense atmosphere 4 billion years ago. Today Mars is a dry, cold arid world where water might exist only on the flanks of large meteor craters and at the poles. The long-term habitability of a planet depends on not-yet-completely understood parameters that include its size, its location, the brightness and activity of its host star, the existence of a magnetosphere to shield its atmosphere, the presence of one large moon to stabilize its inclination, and the history and evolution of the planetary system which may have undergone drastic changes such as collision, close encounter and ejection of exoplanets.
To determine if an exoplanet is inhabited, or simply characterize its basic properties such as temperature, age, and atmospheric composition, astronomers can not rely on the techniques described above but rather have had to develop new ones to extract more information about the exoplanet and its atmosphere.
When a planet is transiting its star, the atmosphere that surrounds it will also transit this star partially attenuating its flux because of absorption from gas molecules, dust and clouds in this non-perfectly transparent exo-atmosphere. We use spectroscopy to spread the light coming from an astronomical object, like a star or a planet, to derive the composition of their atmosphere by identifying absorption lines in the spectrum. Transit spectroscopy is a way to constrain the composition of an exoplanet’s atmosphere by observing a transit and studying the chemical fingerprints of the gases in the planet’s atmosphere.
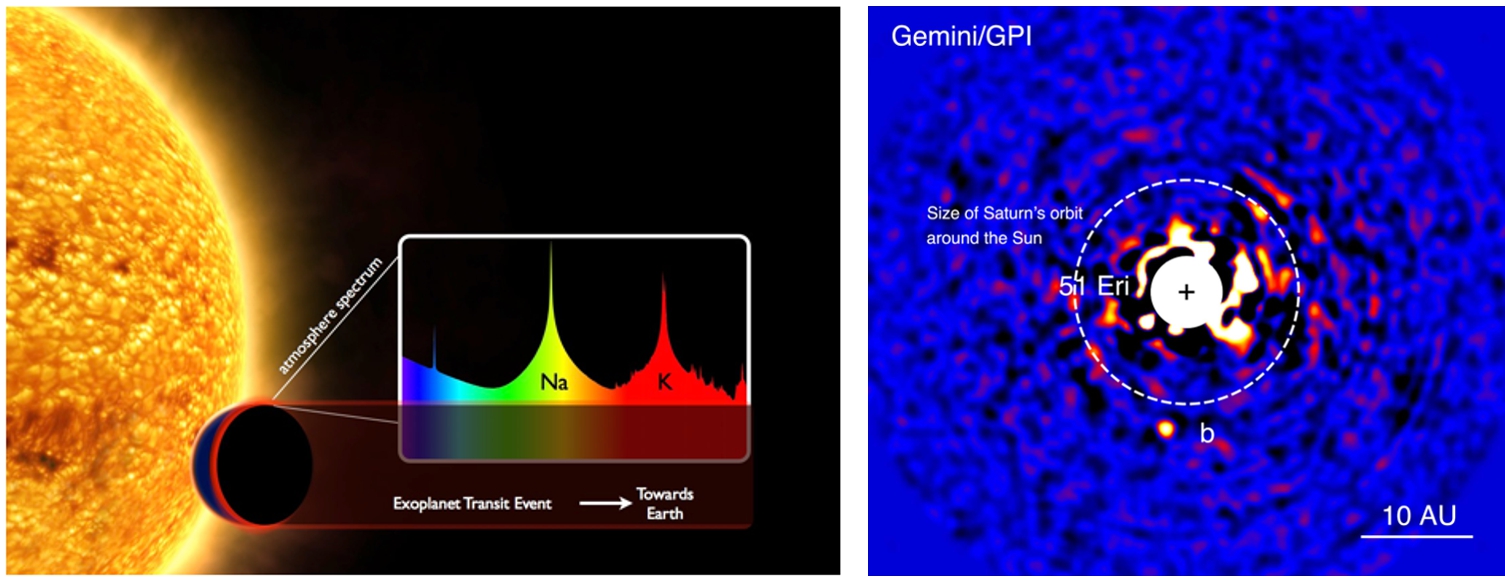
Because the signal is faint due to the relatively small size of the transparent atmosphere as compared to the star, large 8-10 m ground-based telescopes are needed to collect enough visible and near-infrared photons. This technique has been successfully used to characterize the atmosphere of several tidally-locked Hot Jupiters, where traces of sodium, potassium, carbon monoxide, water, and exotic molecules like titanium oxide have been seen.
The future of transiting exoplanets
After the success of the Kepler mission, NASA has an ambitious plan to continue to find and characterize exoplanets by transits. TESS (Transiting Exoplanet Survey Satellite) will be the first-ever space-based all-sky transit telescope that will identify exoplanets as small as Earth around the brightest stars in the sky . The mission’s goal is to monitor more than 200,000 stars to detect exoplanets by transit. Scheduled to start operation in 2018, TESS is expected to catalog more than 1,500 transiting exoplanets, including 500 Earth-sized ones. TESS and Kepler use similar techniques to accomplish very different things. Kepler was a statistical mission designed to stare at one field of view to estimate the rate of exoplanets in our galaxy. TESS will look at bright stars, and thereby study the close environment of our galaxy, helping astronomers to build a map of our solar neighborhood and its planetary systems. Thanks to TESS, future astronomers will be able to say which stars around us have exoplanets, their distance to their host star, and their size. In short, TESS will allow us to start building an accurate map of our galaxy, an important tool for future explorers.
Ultimately, TESS will not provide enough information to fully characterize our neighbor exoplanets. To answer fundamental questions about how planetary systems form and evolve, astronomers must expand their spectroscopic studies of exoplanets to include smaller planets and ones that are farther away. To reach this goal, the European Space Agency (ESA) is considering a medium-class science mission called ARIEL (Atmospheric Remote-Sensing Infrared Exoplanet Large-survey), a space telescope that is designed to investigate the atmospheres of several hundred exoplanets ranging in size from Jupiter to Super-Earths. If the mission is approved, it will be operating in 2026, a few years after TESS has completed its catalog of nearby transiting exoplanets. The core output of ARIEL would be a comprehensive catalog of exoplanetary spectra, yielding accurate molecular abundances, chemical gradients, and atmospheric structures of the planets’ atmospheres. For the first time, we will study the weather patterns on other worlds by detecting diurnal and seasonal variations and the presence of clouds.
The Pale Blue Dot Image of Our Generation?
The proverb “Seeing is believing” summarizes quite well the next major revolution in the study of exoplanets which will consist at seeing an exoplanet. However the task of direct imaging those exoworlds is challenging since an exoplanet is at least a million times fainter than its host star and orbits very close to it. Exoplanets could be seen with a large-aperture telescopes equipped with adaptive optics systems, an optical device capable of correcting aberrations introduced by the atmosphere or imperfections in the telescope, as well as a coronagraph to remove the flux of the central star of the observed planetary systems. These technologies have been validated and improved on ground-based telescopes for two decades. Two 8 m class telescopes, both in Chile, the Very Large Telescope and the Gemini South Telescope, have been equipped since 2015 with an instrument dedicated to the detection and characterization of young Jupiter-like exoplanets. One of the most important discoveries in astronomy in 2015 was made with one of these instruments: the Gemini Planet Imager directly imaged 51 Eridani b, the first exoplanet similar to our own Jupiter . With a mass of twice that of Jupiter, 51 Eridani b is the smallest exoplanet reported among the forty-four directly imaged exoplanets in the NASA Exoplanet Archive. Most of those “seen worlds” are in fact brown dwarfs, or small stars that are not massive enough to sustain nuclear fusion of hydrogen in their cores, and have a mass more than thirteen times that of Jupiter. What astronomers saw in 2015 is the light coming from an exoplanet twice the mass of Jupiter orbiting a star similar to the sun. Their analysis using spectroscopy revealed that 51 Eridani b atmosphere is very similar to Jupiter with water, methane, bands and belts.
Astronomers are bringing us ever-closer to a future filled with directly imaged exoplanets by designing space telescopes specially built to collect images and spectra able to determine the orbits of exoworlds, their temperatures, compositions and, potentially, find fingerprints of life in their atmospheres. WFIRST (Wide Field InfraRed Survey Telescope), a NASA 2.4 m class telescope, is one of them. One of its instruments will be a coronagraph that will give astronomers the opportunity to observe and characterize planets like those in our solar system located from 3 to 10 AU from their parent star. Most of those planets will be characterized using a spectrograph in the visible spectrum that will permit the detection of features like methane, water, and metals in the atmosphere, and easily distinguish between different classes of exoplanets (Neptune-like to Jupiter-like). Scheduled for launch in mid-2020, WFIRST will be the first space telescope equipped with adaptive optics capable of seeing fifty exoplanets similar to those in our solar system and study their properties, including their atmospheres and their surfaces.
What about finding a planet like Earth? If the challenge of imaging Jupiter and Neptune-like exoplanets from space is difficult, imaging a planet like Earth around a star like our sun seems insurmountable. The exoplanet will be in this case ten to 100 billion times fainter than its star and extremely close to it. The good news here is that NASA is already thinking about the next generation of space telescopes with two ambitious concepts on the drawing boards - HABEX and LUVOIR - which would allow astronomers to detect biomarkers, or fingerprints of a biosphere, on distant Earth-like worlds. It is unclear if we yet have the technical knowledge to build and operate a large 8-16 m aperture telescope in space equipped with an adaptive optics, but we know that the design, construction, and launch of these telescopes will take several decades and be extremely costly.
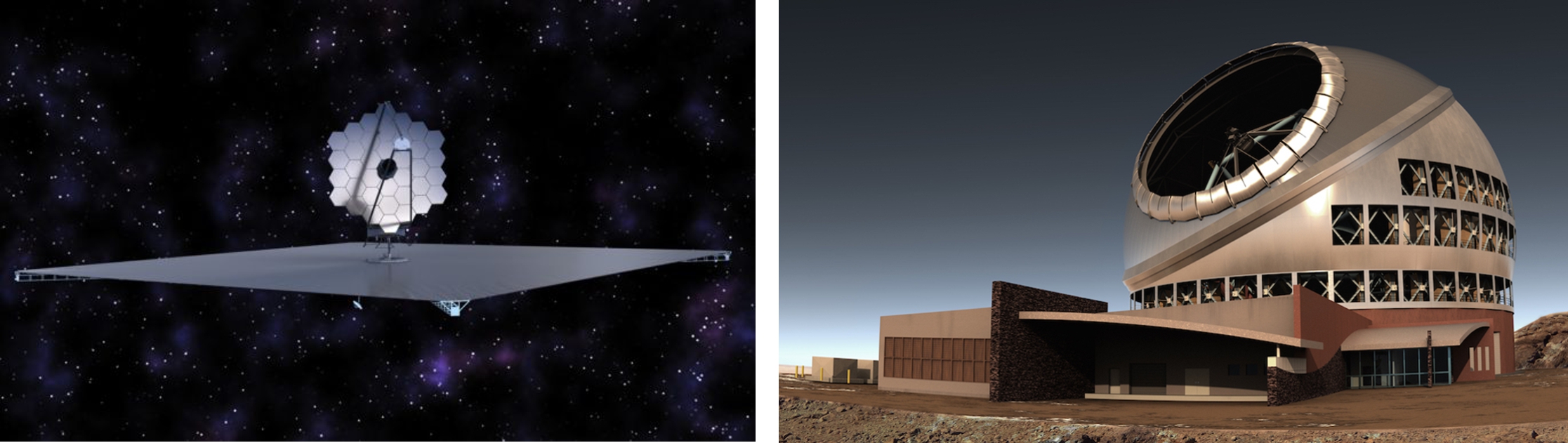
Ground-based astronomy is also evolving quickly, with several giant telescopes currently in construction (TMT, E-ELT, GMT). Because of their large apertures (>30 m) they could have the sensitivity and resolution needed to image and characterize Earth-like exoplanets, especially around stars near our sun. A team of astronomers is pushing for the development of an instrument specifically dedicated to detection of an Earth-like world around Alpha Centauri, a binary star system located at 4.4 light-years from us. Their simulations of the instrument, named TIKI, based on realistic assumptions about its technology revealed that a direct imager in the mid-infrared wavelength range could indeed show the existence of a twin of Earth around one of these stars as early as 2024.
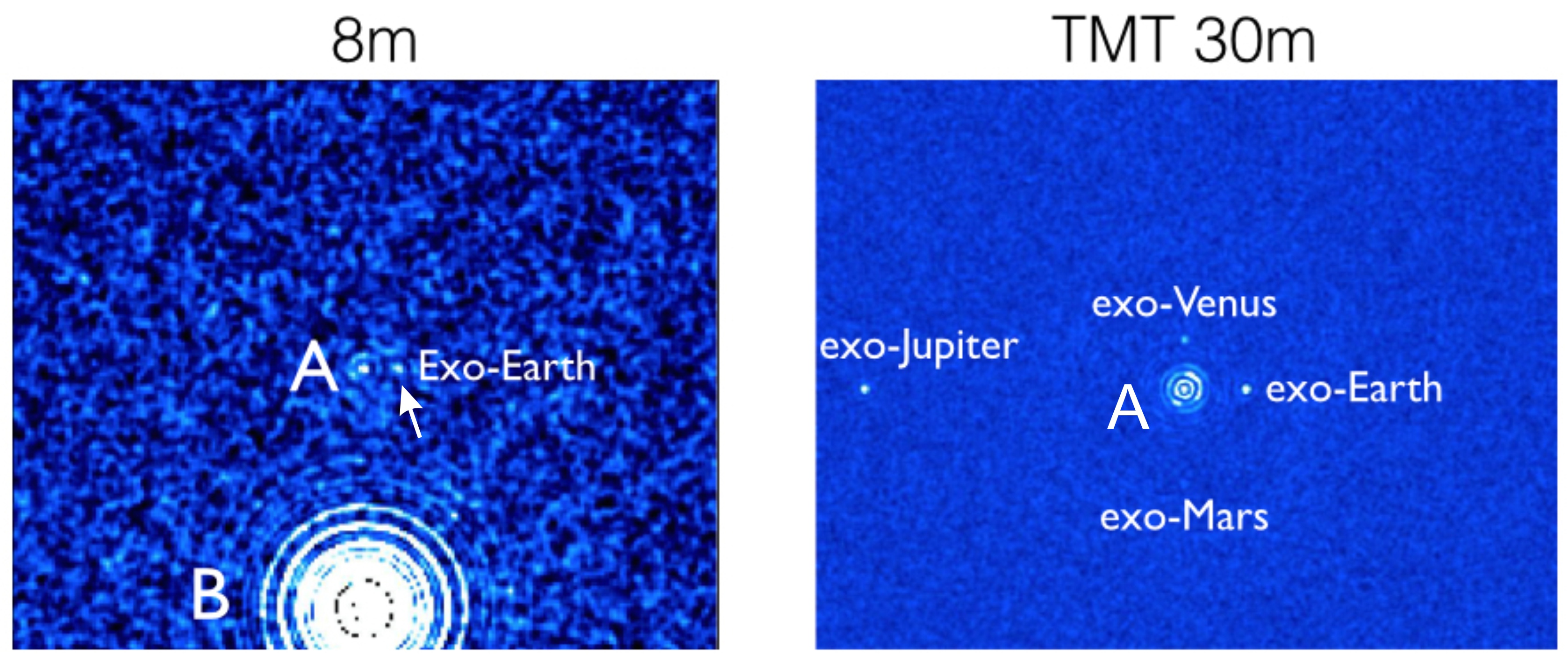
We can’t predict if the first image of an Earth-like exoplanet will be acquired from the ground in one decade, or from space in three decades. It is even less possible to predict exactly what we will see, since nature tends to surprise us.
But I am optimistic and really think that the generation now inhabiting our own Pale Blue Dot will be the one that answers this ancient question: are we alone in this vast universe?
Beyond the science facts, my personal view is that the answer we will get is no, we are not alone. And as that answer sinks in, we will know that we have just received the most important piece of news in the history of our species and our planet.
Responses