Pluto: the first TNO
Trans-Neptunian objects (or TNOs for short) are a collection of minor planets that orbit the Sun at a distance greater than 4.5 billion km (2.8 billion miles). TNOs are some of the most recently discovered objects in our solar system, with the first one, Pluto, being discovered in 1930 (Figure 1); other well known TNOs include Eris, Makemake and Haumea (Figure 2).
In the early 1900s there was believed to be a discrepancy in the orbits of Neptune and Uranus predicted to be due to another (undiscovered) distant object. The search for this object led to the discovery of Pluto! However Pluto was too small to cause such a discrepancy and it wasn’t until Voyager 2 flew past Neptune in 1989 that the mystery was finally solved. Perturbations on the spacecraft provided a way of accurately determining the mass of Neptune, which was shown to be 0.5% less than originally thought - fully explaining the discrepancy!
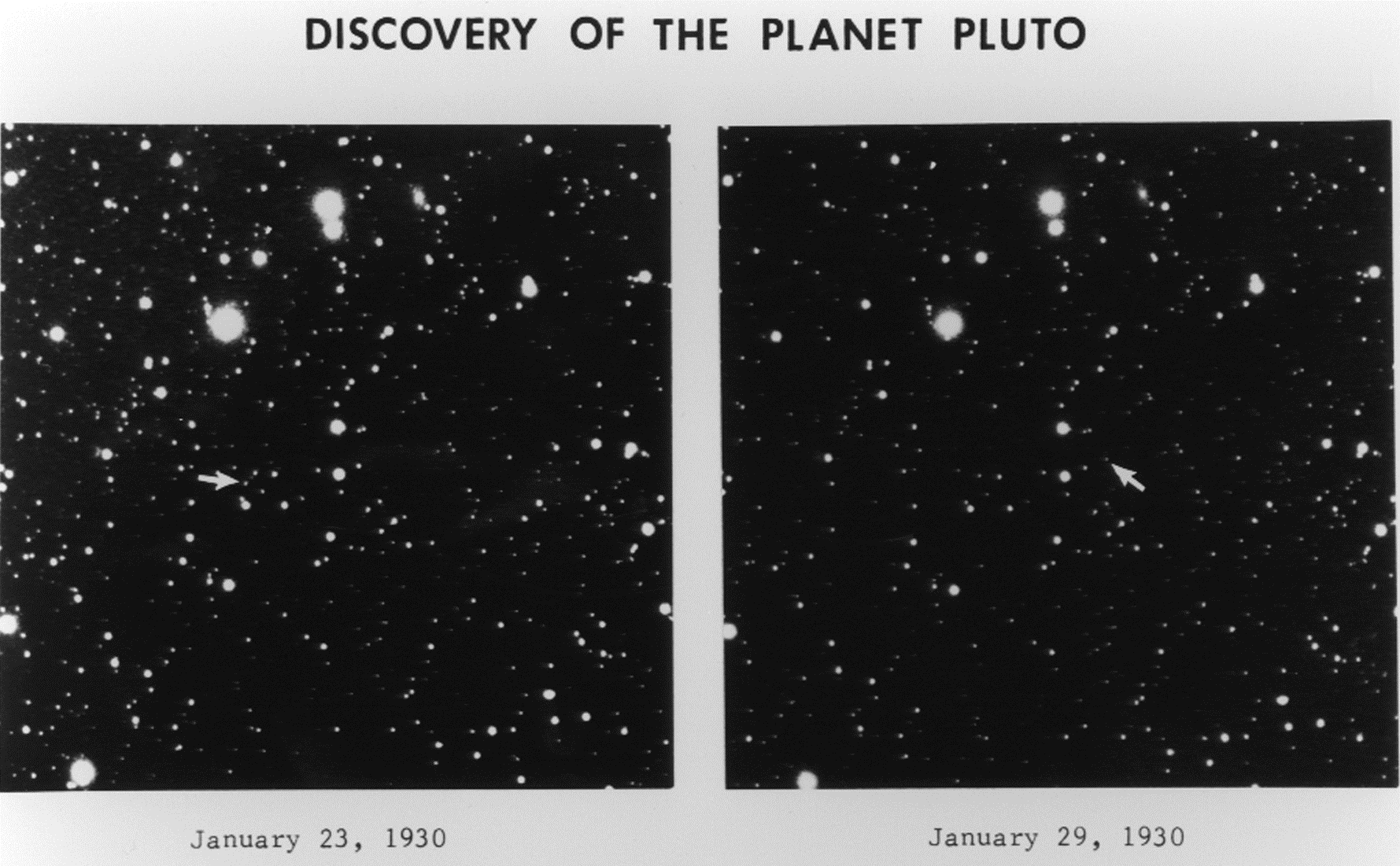
Pluto’s discoverer, Clyde Tombaugh, continued searching for other TNOs but was ultimately unsuccessful, leading to the notion that Pluto was alone in the distant solar system. However, in 1992 another TNO was discovered (15760 Albion), which prompted a broad sky survey in which hundreds of TNOs were discovered. In 2005 Eris was discovered, a TNO more massive (but smaller in size) than Pluto. The combination of the discovery of a large population with similar orbital dynamics to Pluto and Pluto not being even the most massive of these objects called into question Pluto’s status as a planet. In 2006 the IAU declared Pluto (and Eris) to be dwarf-planets. This change, seen as a demotion by many, continues to be controversial - promoting fierce debate on both sides .
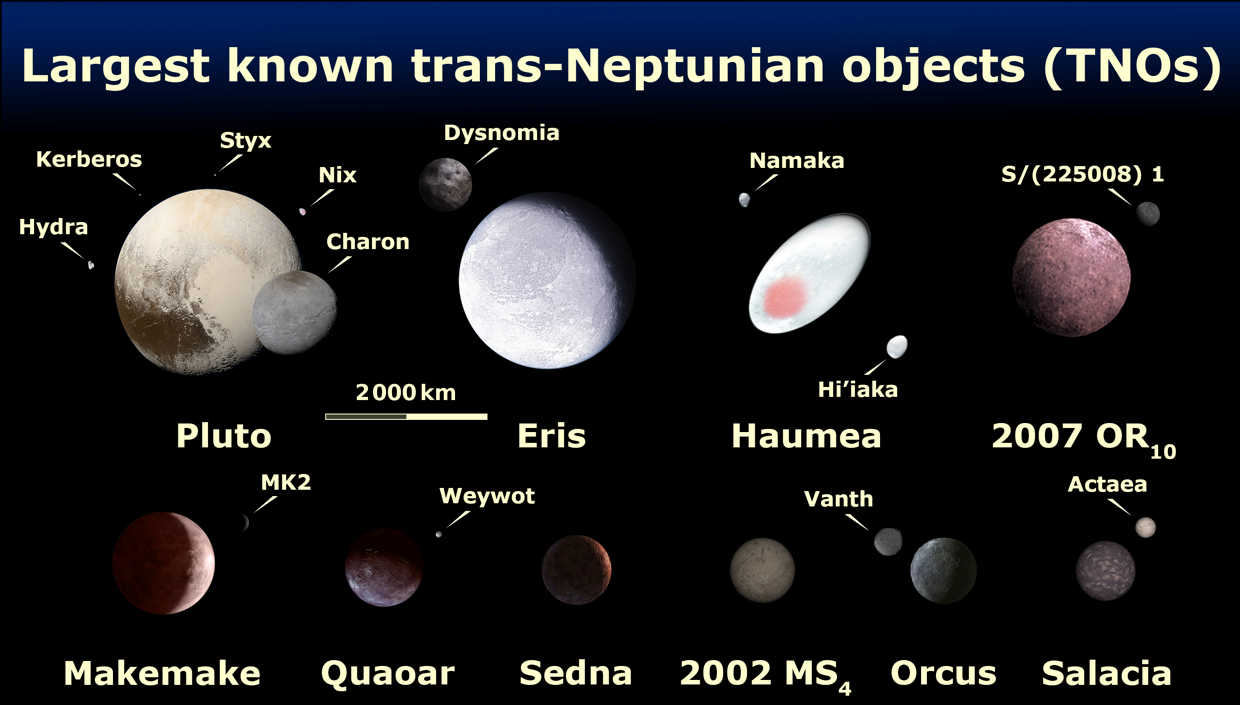
A large number of TNOs are presently known to exist: 528 numbered, and around 2,000 un-numbered objects (objects become numbered by the Minor Planets Center when their orbits are well understood), along with 80 satellites (i.e. moons) of TNOs. Investigations have shown that this population can be split into two sub-categories based on their distance from the Sun and other orbital parameters: Scattered Disk Objects and Kuiper Belt Objects. These sub-categories (as shown in Figure 3) are important as they allow scientists to determine which objects are alike and whether any are unusual. Let’s briefly consider these categories one at a time.
Scattered Disk Objects and the "Planet Nine" Hypothesis
Scattered disk objects (SDOs) orbit further from the Sun than Kuiper Belt Objects, having a mean distance from the Sun of >55 AU and are in very eccentric and inclined orbits (i.e. non circular and tilted). SDOs can also be further categorized into: extreme TNOs (eTNOs), typical scattered disk objects and detached objects.
eTNOs are the TNOs most distant from the Sun (with a semi-major axis of between 150 and 250 AU). The improbable alignment of some eTNOs, which have their points of closest approach of the Sun clustered in one direction and similar orbital tilts, has led to the hypothesis that a large, undiscovered planet is shepherding their orbits . This planet is predicted to be a super-Earth (10 times the mass of the Earth, with a diameter 2-4 times that of the Earth). There are many ideas as to such a planet’s origin: perhaps it is an ejected core from a once giant gas planet of our solar system, or a planet captured from another solar system. However, other hypotheses to explain the observations also exist: one alternative explanation is that there is actually no alignment of eTNOs, but rather we are observationally biased to find objects that have this type of orbit. Furthermore, since only a small number of eTNOs have been discovered it makes an accurate statistical analysis of the population difficult to achieve. Thus, perhaps a combination of observational bias and small number statistics makes it appear there is clustering in the orbits of some eTNOs when really there is none .
Other alternative explanations include the hypotheses that the clustering is real, but caused by a distant belt of material (i.e. not a single object ), or a larger object even further from the Sun (up to 15 Earth masses located 200-300 AU from the Sun ) or maybe not just one but four objects . At the time of writing none of these hypotheses have been proven, and so the hunt for objects deep in our solar system continues.
Kuiper Belt Objects
Kuiper Belt Objects (or KBOs) are closer to the Sun than SDOs, having an average orbital distance from the Sun between 30 and 55 AU. Their orbits are close to circular and close to the ecliptic. KBOs can be further split into resonant and classical objects, where resonant objects are tidally locked to Neptune whereas classical objects are not. This resonance occurs because (despite their distance) Neptune and these KBOs exert a periodic gravitational influence on each other, which results in their orbits synchronizing.
The resonant population can be further split by the type of resonance they have, those with a 1:2 resonance (i.e. they orbit the Sun once in the time Neptune orbits it twice) are called twotinos, while those with a 2:3 resonance with Neptune are called plutinos (after their most famous member, Pluto). Classical KBOs (sometimes called cubewanos) are also further split between cold-classical objects (that have an orbit with a low, <5 degree, inclination) and hot-classical objects (which despite their misleading name aren’t hot, but rather have a high orbital inclination). Cold-classical KBOs are perhaps some of the most fascinating objects of our solar system, because they are likely unchanged since they formed when our entire solar system was forming about 4.5 billion years ago. Recent exploration of the Kuiper Belt by NASA’s New Horizons spacecraft has led to a much better understanding of both Pluto and a classical KBO.
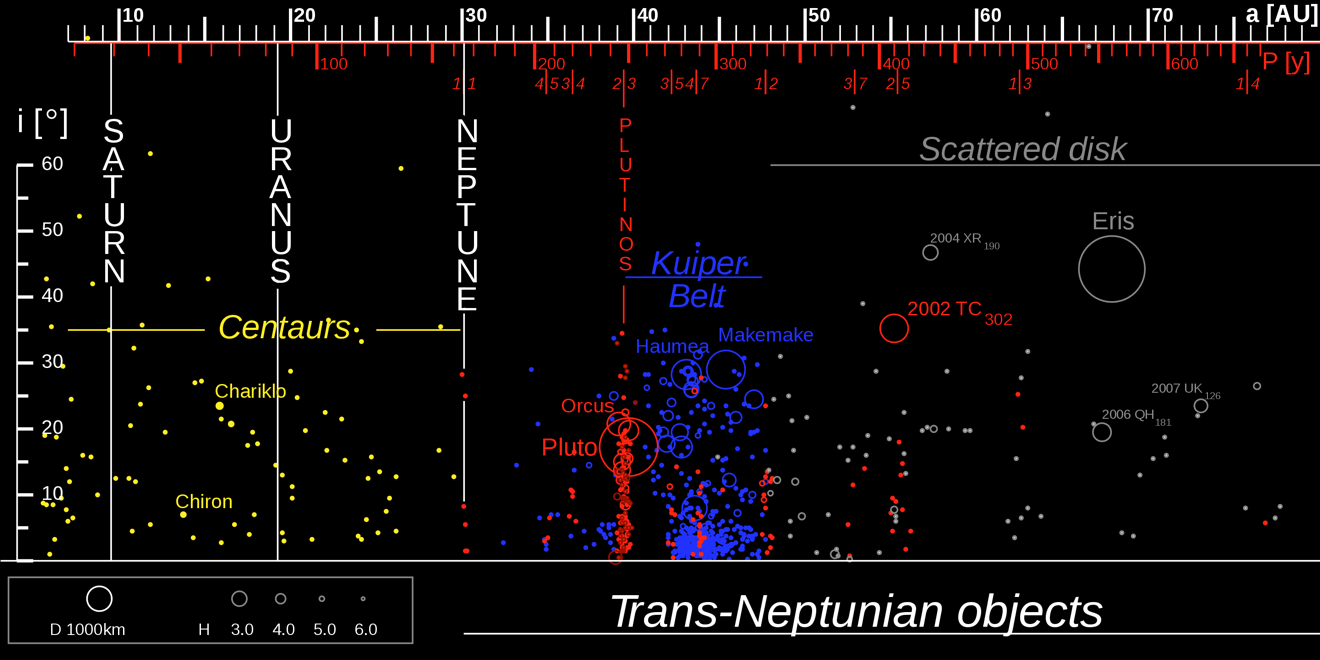
NASA's New Horizons space mission
In-situ exploration of TNOs has been led by NASA’s New Horizons mission, which flew past Pluto (the most famous KBO) in 2015 and onto MU69 (a cold classical KBO) in 2019. New Horizons revolutionized our understanding of these worlds. Prior to New Horizons’ arrival all that was known about Pluto was gleaned from it being a small dot of light and its interaction with its large moon Charon, and MU69 wasn’t even discovered when New Horizons launched back in 2006.
The Pluto system
We knew from measurements made using the Hubble Space Telescope (HST) that Pluto’s surface wasn’t uniform in color and albedo, implying a non-uniform composition. There were models of Pluto’s surface that implied it could be warm enough to support an atmosphere, and volatiles on the surface could be warm enough to sublimate into the atmosphere in warm regions, and freeze out from the atmosphere in cold one. However, all of this could not prepare us for what New Horizons revealed (see Figure 4)! Pluto has active geology in its bright heart-shaped basin known as Tombaugh Regio. The two lobes of the heart are geologically distinct, with the western lobe (Sputnik Planitia) being smoother and less blue. In Sputnik Planitia nitrogen ice (and a small amount of carbon monoxide ice) slowly overturns, renewing its surface. This region is so new not a single crater can be observed on it, making it impossible to age but implying it has a very young surface (less than about 10 million years). This is astounding given that Pluto has little external energy to power such activity: it is far from the Sun (at its closest its 29.6 AU away), is small (meaning it might not have been big enough for it to support substantial radiogenic heating; its radius is 1,188 km - making it less wide than the continental USA), and doesn’t have a large gas giant near it to provide tidal heating, such as is exemplified by Jupiter and its four main moons. However, it is believed that either Pluto’s interior is providing enough radiogenic heating to mobilize these ices, or direct solar insolation is enough .
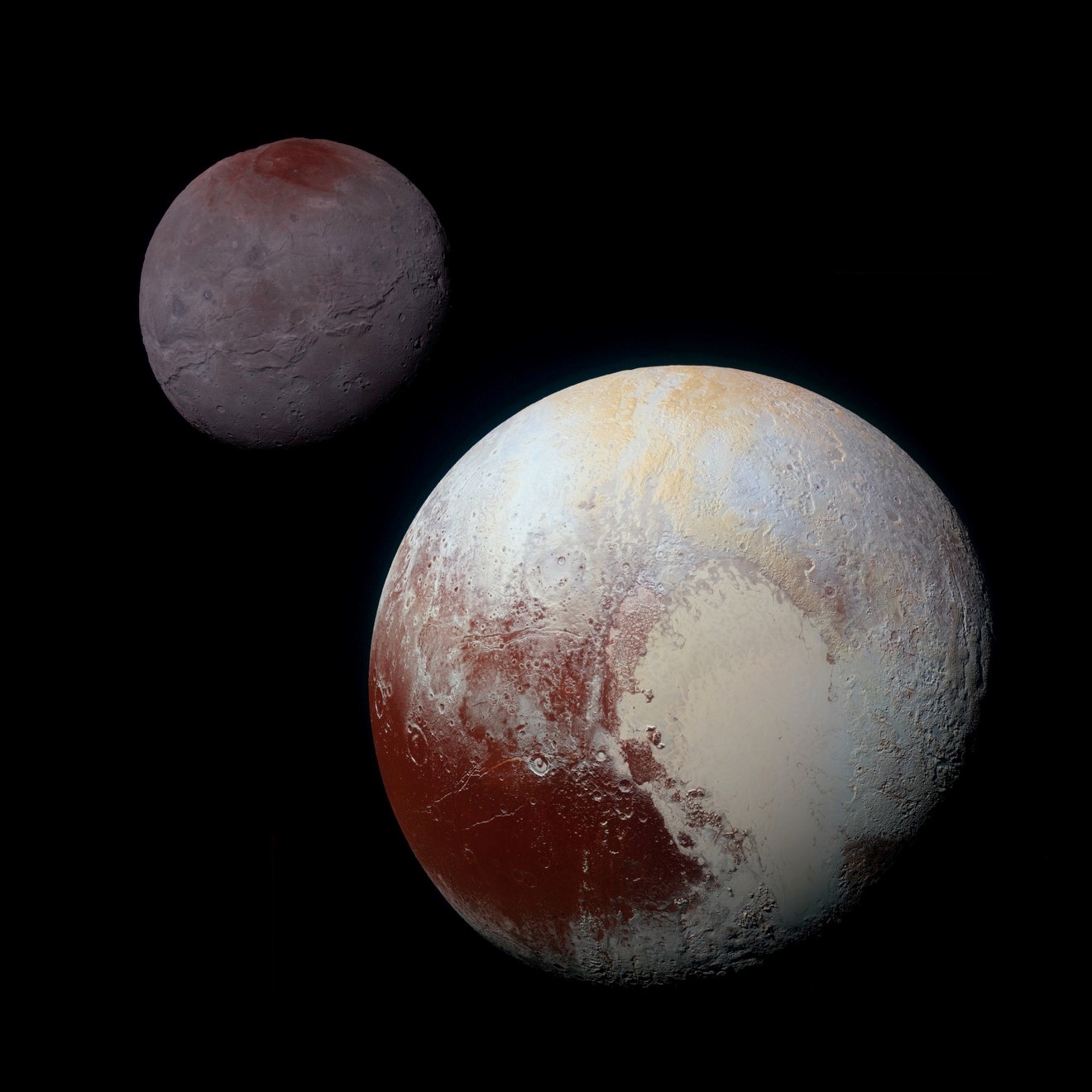
Perhaps in the past Pluto was even more active. There are two intriguing features south of Sputnik Planitia near Pluto’s south pole provisionally named Wright and Piccard Mons (see Figure 5). They are the tallest peaks on Pluto (~4 km high, ~150 km across) and have a large depression at their summit and hummocky flanks, leading to the hypothesis that they might be cryovolcanoes. No evidence of cryoactivity was observed at the time of the New Horizons encounter. However, the features appear lightly cratered, which implies they are geologically young (but not as young as Sputnik Planitia). They are the first large potentially cryovolcanic constructs to have ever been observed.
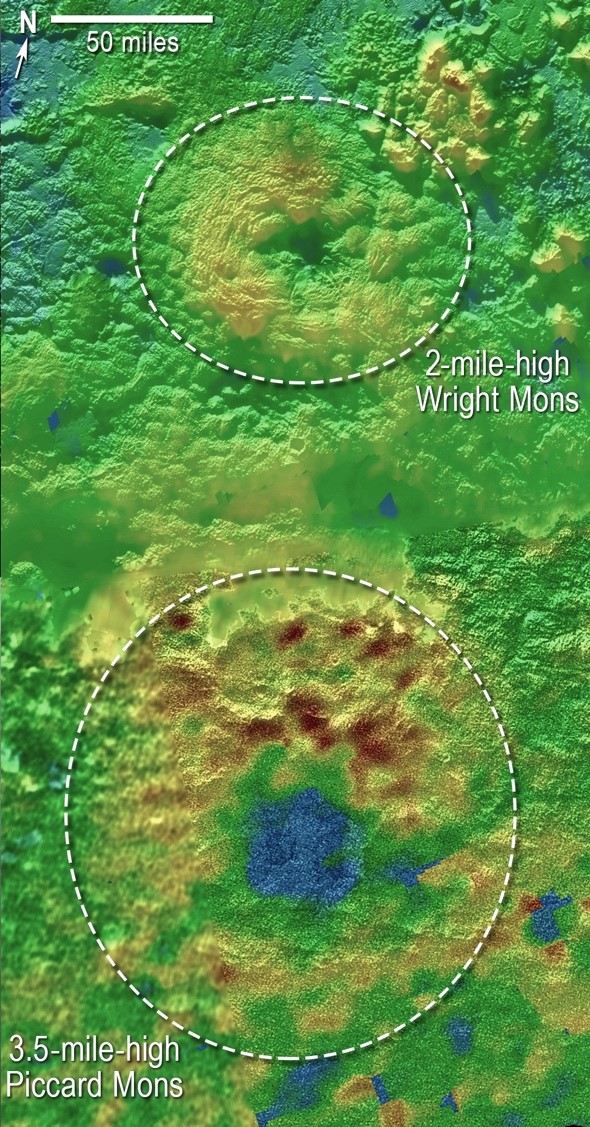
The rest of Pluto’s surface doesn’t disappoint either, from the penitente covered Tartarus Dorsa, to the dark red band of Cthulhu Macula, to the methane ice covered northern polar cap. However, it's what’s above the surface that arguably provides some of the most breathtaking views: images returned by New Horizons show Pluto has an atmosphere, making it the only known KBO to have one! Images of Pluto in eclipse show a wonderful blue haze during sunrise/sunset (Figure 6), whereas the banded structure is more clearly seen in the black and white images taken just after closest approach (Figure 7) .
Pluto’s atmosphere is made up of nitrogen, methane and carbon monoxide and has a pressure around 100,000 times less than Earth's. The atmosphere is supported by the sublimation of volatiles from its surface. There are at least twenty haze layers in the atmosphere, extending above 200 km from the surface. The hazes are probably different types of heavy hydrocarbons, formed from the interaction of cosmic rays with Pluto’s gaseous atmosphere .
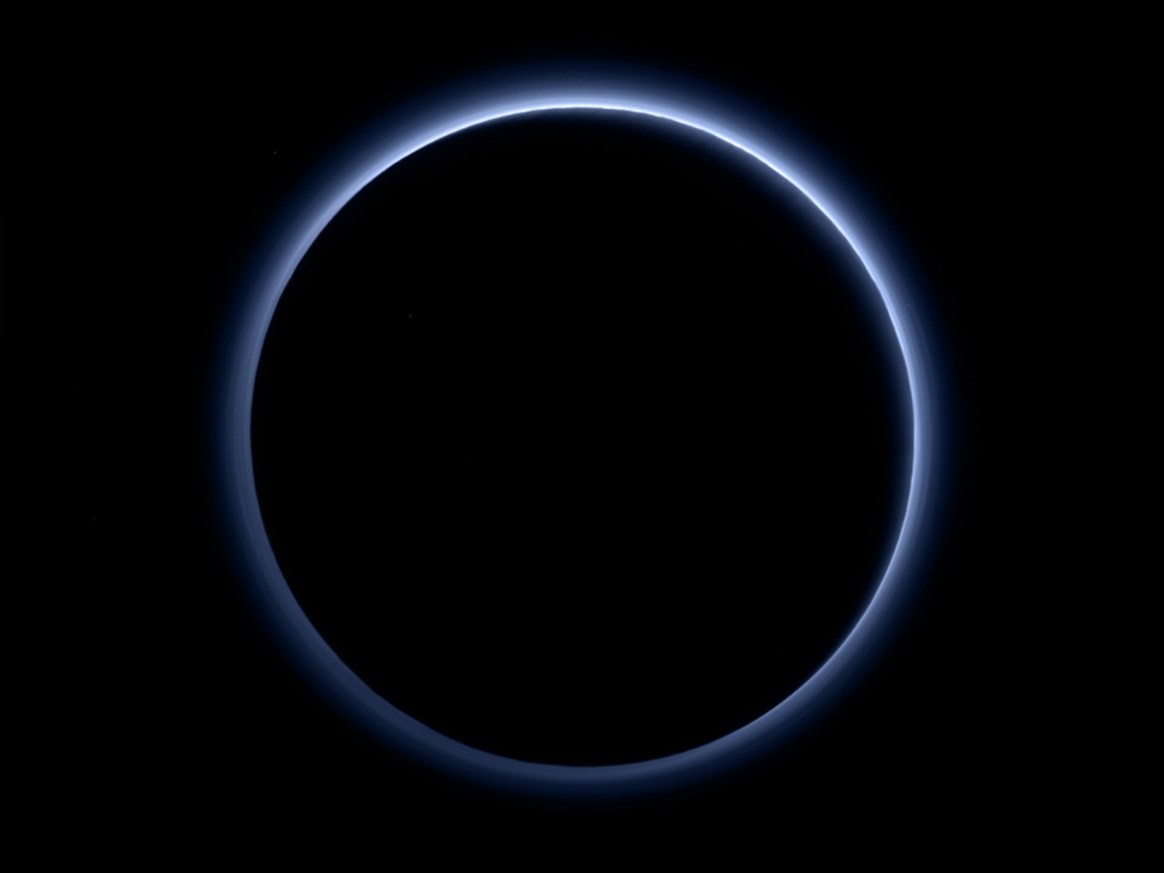
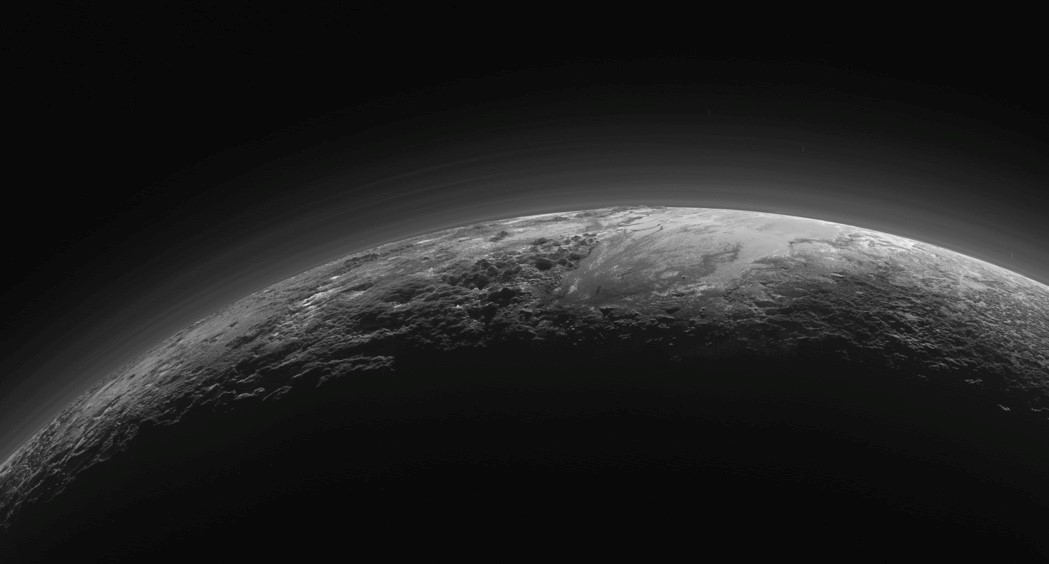
Of course New Horizons did not just observe Pluto, but rather it viewed the entire Pluto system, including Pluto’s large moon Charon and its four smaller moons. Possibly the most surprising result was the dark red color on Charon’s northern polar regions, thought to be due to the capture, cold trapping, and subsequent processing of Pluto’s atmosphere onto Charon’s surface (Figure 4) .
All four of Pluto’s small moons (Styx, Nix, Kerberos and Hydra, shown in Figure 8) have prograde nearly circular orbits in the same orbital plane as Charon. However, they are all much smaller than Charon (Nix and Hydra are about 42 and 55 km across each, while Styx and Kerberos are 7 and 12 km respectively). Color images of these moons were difficult to obtain (due to their small size), and only the larger satellites (Hydra and Nix) were successfully imaged in color. Hydra was the opposite side of Pluto from New Horizons during encounter, and so its color images are poorly resolved. However, Nix was successfully imaged, and shows a grey surface with a red region around a large crater. It is not known whether the impactor was red, or whether it excavated Nix’ surface which either was redder to begin with, or turned redder with exposure to the radiation of space .
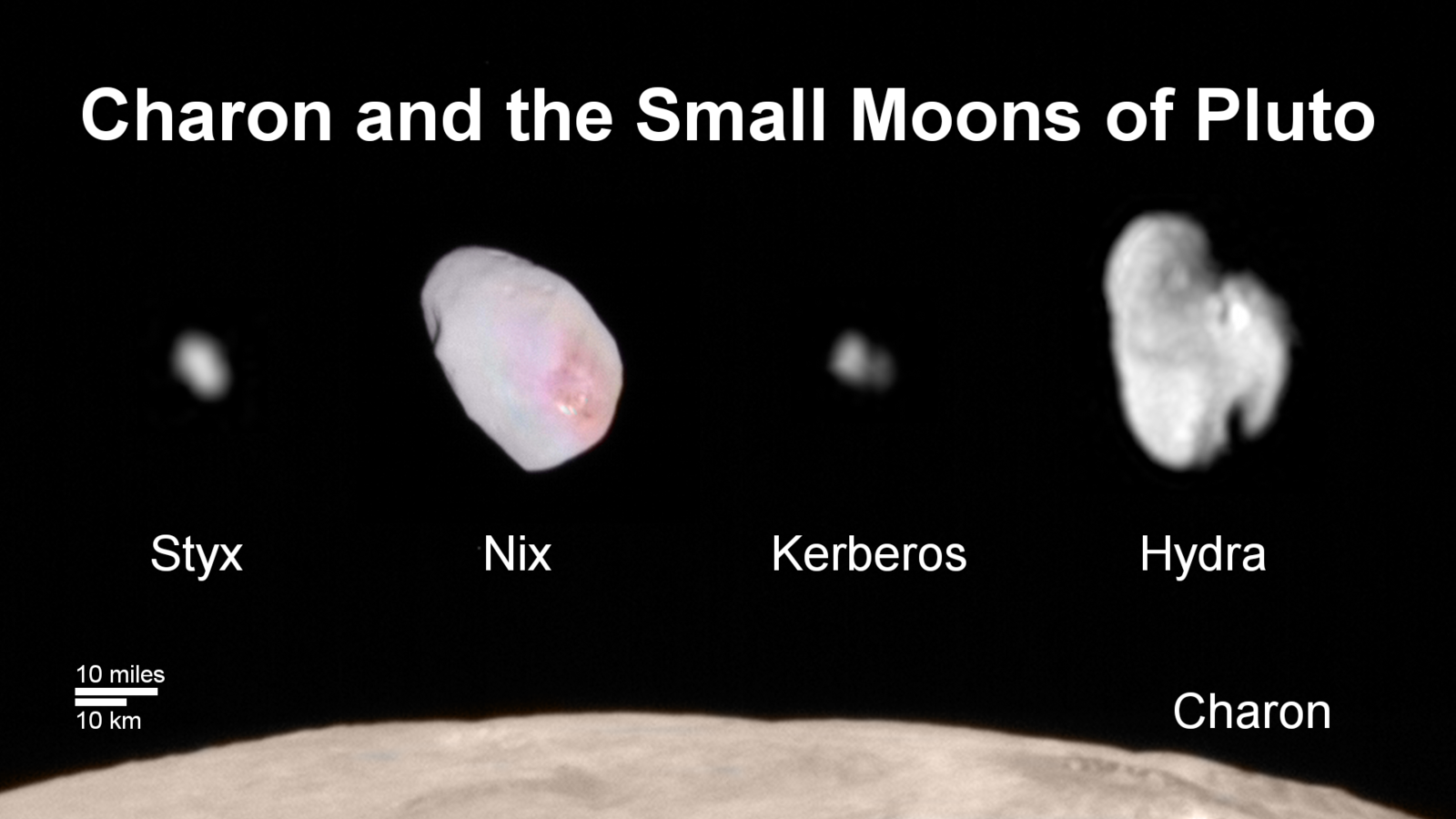
MU69 - Ultima Thule
Recently, in January 2019 New Horizons went on to explore (486958) 2014 MU69 (sometimes nicknamed simply MU69, or Ultima Thule). MU69 is a very different type of TNO from Pluto: a cold-classical KBO. MU69 is both the most distant object ever explored (at 44.6 AU) and the most primitive; it is likely unchanged since its formation billions of years ago, and is providing unprecedented insight into how our solar system, and even how our own planet formed.
The images taken during the recent encounter are still trickling down from the spacecraft at 1-2 kilobits per second (think 1990s dial up speed), but the initial results (see Figure 9) show it to be a fascinating world. It is a contact binary ~31 km long (slightly smaller than central London), and is made of two touching unequally sized lobes 19 km and 14 km (imaginatively) nicknamed Ultima and Thule. Both lobes appear lumpy, dark, and uniform in color except in the neck region that links the lobes, which appears brighter and less red.
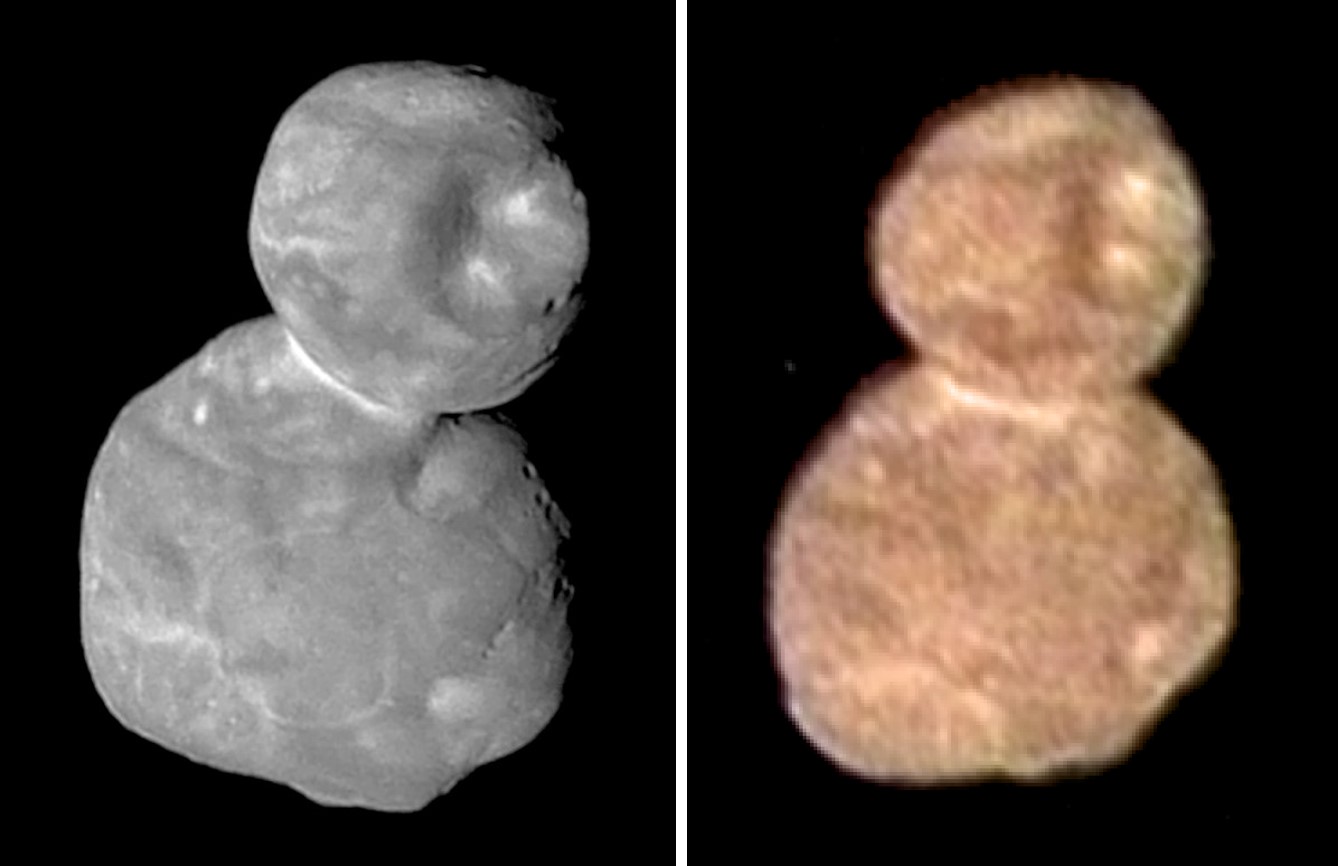
This is the first time the raw, unchanged, building blocks of a planet (known as a planetesimal) has been seen. MU69’s bi-loped lumpy appearance points to it being made from two objects that came together, probably at very low speeds (walking pace). They don’t have a large gravity, and so a higher-speed impact would have destroyed, sent them moving away from one another, or some mixture of these two outcomes. Each lobe formed separately from pockets of dust in the early Sun’s rotating protoplanetary disk that were gravitationally attracted to one another. These clumps were able to move through the gas disk to form larger clumps, which would have attracted even more material, a process sometimes referred to as pebble cloud collapse.
Our understanding of the secrets MU69 holds for us is just beginning. To date only a small fraction of the images and other data New Horizons took during its encounter has been downlinked and analyzed, and the first science papers are only just being written! The highest-spatial resolution ones and spectral information are still to come! These data, with updated formation models, will tell us MU69’s shape, color, composition, along with its implications for its own evolution and by extension every body in our solar system!
Conclusion and outlook
The elusive and hard-to-study nature of TNOs makes them some of the most intriguing places in the solar system. Understanding TNOs has pushed the limits of astronomy for almost a century, and recent instrumentation (e.g. Hubble Space Telescope) and missions (e.g. New Horizons) have provided unprecedented insights into these worlds. They have shown us everything from active geology to ancient unchanged surfaces. New instrumentation and future missions will build upon this work: one instrument of particular note is the Large Synoptic Survey Telescope (LSST), which is expected to have first light in early 2020. The LSST will photograph the entire available sky every few nights with the expectation that another 40,000 TNOs will be discovered, and their orbits, colors and shape characterized. All of this information will provide further insights into the formation and evolution of our solar system.
Want to know more?
- http://pluto.jhuapl.edu/
- Stern A and Grinspoon D (2018) Chasing New Horizons: Inside the Epic First Mission to Pluto Picador
- Stern SA, Grundy WM, McKinnon WB, Weaver HA, and Young LA (2018) The Pluto System After New Horizons Annual Review of Astronomy and Astrophysics 56: 357-392
Responses