Cephalopod Behavior: Camouflage, Trickery, and other Curiosities
By Anne-Sophie Darmaillacq and Ludovic Dickel in
Could Victor Hugo have imagined two centuries ago, when in Toilers of the Sea he related the fight between Gilliatt the Guernsey fisherman and the sea monster, that octopuses and their relatives (cuttlefish, squid and nautiluses) would have been considered smart, sentient creatures by European citizens? Yet this has been the case since the 1st of January 2013, when the new European Directive on the protection of animals used for scientific purposes came into effect. This EU directive (2010/63/EU) includes and recognizes cephalopods, which are molluscs just like snails and clams, as sentient animals: a world premiere for invertebrates!
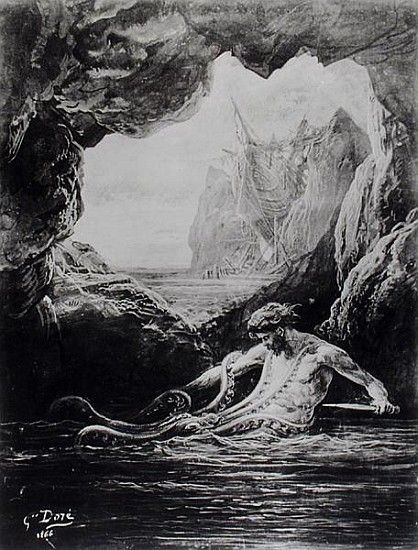
Although cephalopod behavior has been a focus for researchers since the 1950s, only a few of the hundreds of cephalopod species have been extensively studied, mainly cuttlefish (Sepia species) and octopuses (mainly Octopus vulgaris). Pioneering work first investigated octopus brains and processes involved in their learning and memory. More recently, attention has turned to the ability of cephalopods to change their body colorations and patterning, and to their visual systems. Although much more research is required, it has become clear that this group of animals is amazingly intelligent and that their neural abilities rival those of vertebrates in terms of innovation, deception, learning and memory. Among invertebrates cephalopods are most likely the group with the richest behavioral repertoire.
From the smallest (Idiosepius, approximately 5 mm) to the largest (Architeuthis sp., the giant squid, more than 18 m long including tentacles, and Mesonychoteuthis sp., the colossal squid, about 10 m long including tentacles and weighing up to 500 kg), cephalopods are exclusively marine; they are broadly distributed and are found in coral reefs, in the ocean depths, and in polar waters. They feed mostly on crustaceans (shellfish, crabs, shrimps) and fish, while they themselves are coveted prey for a variety of fish, marine mammals and sea birds.
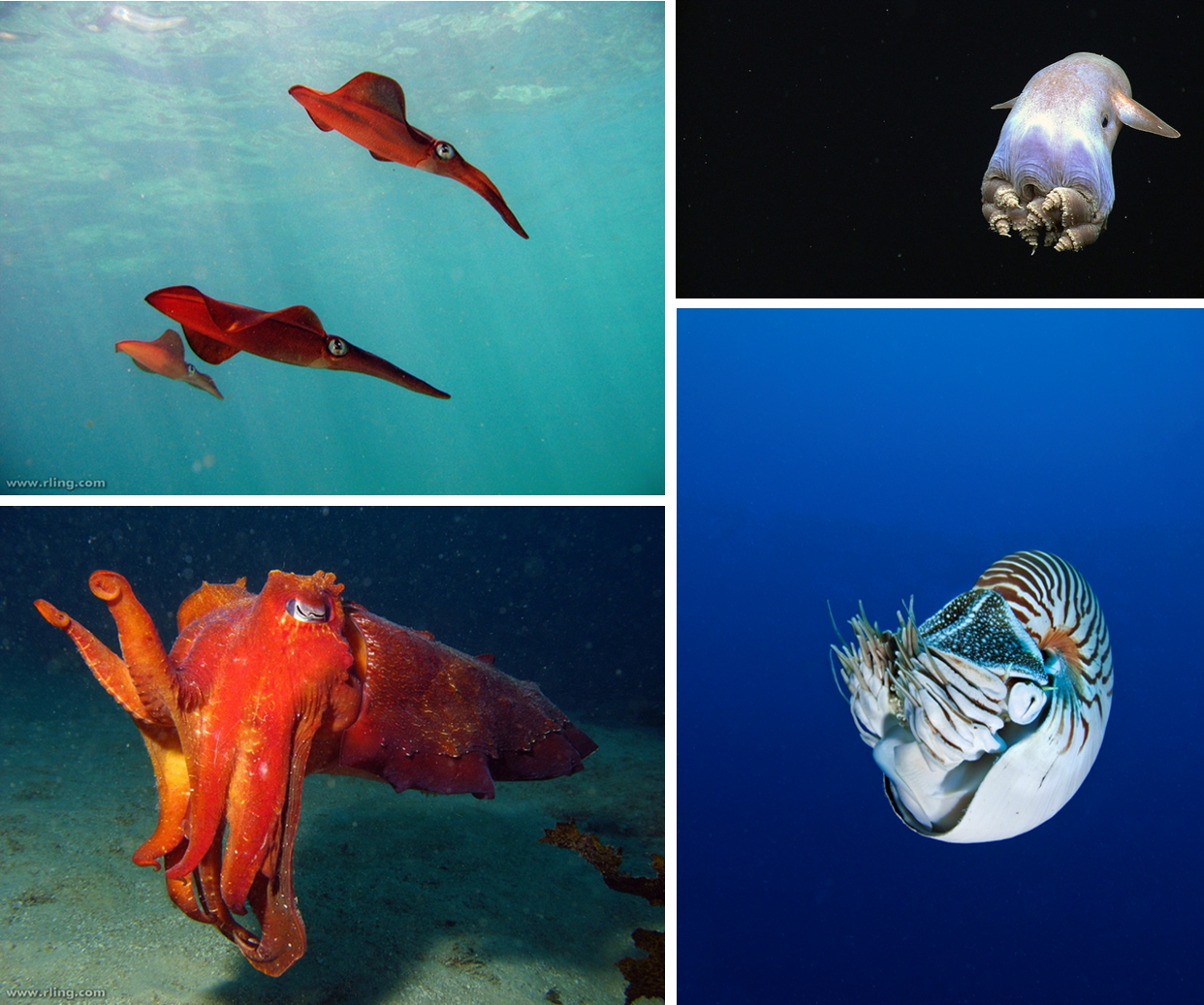
A brain in a soft body
During their evolution cephalopods lost their ancestral external molluscan shells and developed arms and suckers. Their skin can change color instantaneously thanks to direct neural control. They are efficient swimmers and propel themselves using a powerful jet of water from a ventrally situated flexible funnel. Squid and cuttlefish also use their fins to fine-tune their movements. All appendages (arms, suckers, and fins) are controlled by a large brain. The cephalopod brain is the most advanced of all invertebrates, consisting of 300 to 500 million neurons compared to 90 thousand in their snail cousins, or 950 thousand in a very smart bee. Strikingly, an octopus has more neurons than a rat or a mouse, but it is difficult to compare a mammalian brain with that of a cephalopod because of a fundamentally different brain organization.
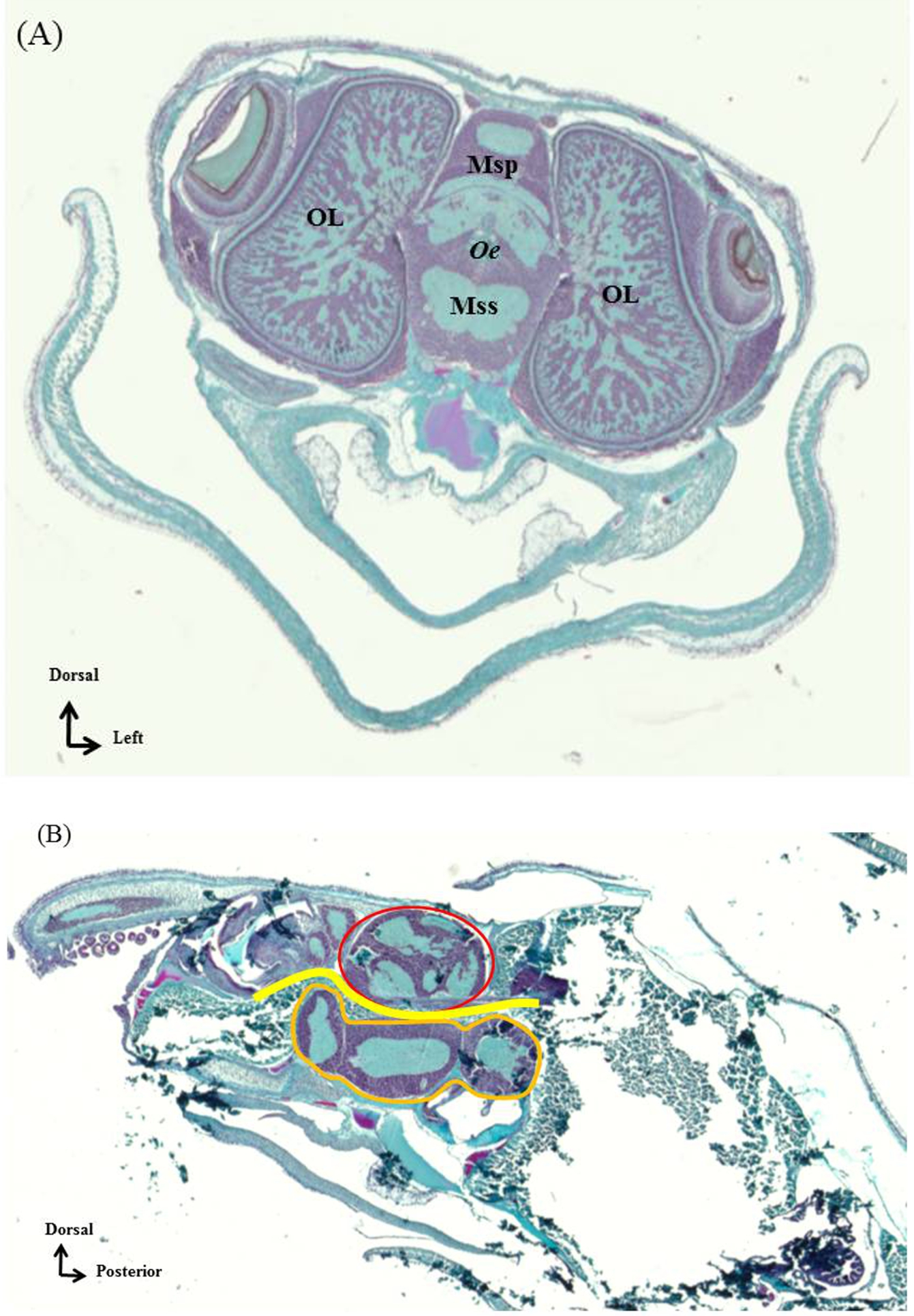
The cephalopod brain is situated between the eyes, protected by a cartilaginous “cranium” and distributed around the esophagus. The ventral and intermediate parts of the brain are associated with sensori-motor integration and control movements, while the most dorsal part of the brain is probably involved in higher-order neural processes including associative learning, memory formation, and decision making. Laterally and aligned with the eyes are two optic lobes, which are responsible for visually guided behavior (prey detection, changing of color patterning). The optic lobes typically represent two thirds of the entire brain volume, which underlines the great importance of visual information in cephalopod behavior.
Body coloration
Body coloration (either homochromy or disruptive coloration) is very important to cephalopods for concealment within their environment, for deceiving a predator by mimicking a dangerous or poisonous animal, for threatening a competitor, or for attracting a potential mating partner. The capacity to change body pattern in a flash for all of these purposes is one of the main characteristics of cephalopods, and is facilitated by specific cells within the skin. The deep layer of their skin is uniformly white. On this canvas hundreds of thousands of pigmented cells (the chromatophores with yellow, orange-red and brown-black pigments) can contract or expand, thereby changing the entire body color or drawing contrasting geometrical figures on the back of the animal (disruptive coloration). To complement these extraordinary paintings, a layer in the skin contains iridophores that act as millions of tiny mirrors and reflect the incoming light in different directions. These cells produce iridescence, which is highly conspicuous in a marine environment. The combination of these three types of cell (white cells, chromatophores, and iridophores) allows octopuses and cuttlefish to display a range of optical effects (as seen, e.g., in blue ringed octopus or flamboyant cuttlefish). In combination with postural changes a squid, a cuttlefish, or an octopus can disappear almost completely from sight within a second by imitating the substrate, algae, or rocks (imitation or homochromy).
When detected and threatened by a predator, cuttlefish, squid and octopuses can display a frightening coloration by drawing two big spots (“false eyes”) onto their white backs; this is called a deimatic pattern. They can also jet black ink to distract and surprise the predator while escaping.
Stimulus-response versus cognition
Are these defensive behaviors only stimulus-response reactions or do they depend on individual cognitive skills? Research with cuttlefish seems to indicate that camouflage does indeed depend, at least in part, on cognitive skills.
Firstly, the development of camouflage is not rigidly fixed. Juvenile cuttlefish reared on variegated backgrounds are better at concealing themselves against new, artificial backgrounds than cuttlefish reared on uniform backgrounds, suggesting that the response to the background is subject to individual experience .
Secondly, depending on the surrounding environment, cuttlefish adjust their skin patterns in relation to their own size. Studies have shown that cuttlefish that are placed on a white and black checkerboard with varying tile sizes adjust their camouflage coloration according to their body size, suggesting that they are perceptive of the dimensions of their own body pattern .
Thirdly, responses to threat are not “all or none” and there are substantial differences in concealment strategy between different individuals, suggesting that defensive behavior is highly plastic and may be a result of complex decision-making processes .
And finally, recent work has shown that cuttlefish can be conditioned to change body patterns in response to rewards, demonstrating the involvement of learning and memory in body patterning .
From these observations, body patterning appears to be the result of complex integration of visual information at different neural levels, including discrimination, learning and memory, self-perception, and decision making. The mechanisms responsible for expressing the diverse body patterns in cephalopods remain poorly understood; fortunately there are increasing numbers of studies being conducted with the aim of identifying low-level mechanisms, as well as possible cognitive aspects, of their camouflage. Intriguingly, the richness of this behavior offers new insights into how these animals perceive their environment and even allows the functioning of cephalopod brains to be directly addressed, just like a sophisticated natural brain imaging system!
Visual specificities
Cephalopods have large, single chambered camera-type eyes whose optics and structure resemble those of vertebrate eyes; this convergence has probably been driven by a shared ecology and competition with fish.
Vision underwater is very different from that in the air. Firstly, light entering water is absorbed depending on its wavelengths: red, orange and yellow colors disappear first, then green colors are lost and, with increasing depth, light becomes weaker and more blue. Secondly, light is scattered by dispersed or suspended particles. Moreover, when sunlight is scattered or passes through transparent objects, it becomes polarized. There is physiological and behavioral evidence that cephalopods are color-blind but sensitive to the linear polarization of light (A recent study, however, has suggested that they are in fact able to perceive spectral information with their specially shaped pupils). Whereas most vertebrates (including humans) are completely blind to polarization, cephalopods seem to master this sensory quality thanks to the special arrangement of the photoreceptor cells in their retinas. Some authors have even suggested that polarization sensitivity in cephalopods could be an analogue of color vision. Polarization sensitivity has multiple functions. It allows cuttlefish, for instance, to break the natural camouflage of silvery fish or transparent shrimps. In line with this observation it has recently been shown that, like insects, cuttlefish can use both polarized and unpolarized cues to reach a shelter in a Y-maze experiment. In the event of conflicting information, they tend to preferentially follow polarized cues for orientation rather than unpolarized cues.
Polarization sensitivity develops during the first month of life in juveniles, and its acuity seems to depend on environmental conditions. European cuttlefish from the English Channel can detect polarized signals from further away and in much more turbid water than Pharao cuttlefish from the Red Sea. Moreover, squid and cuttlefish are known to reflect linearly polarized light from specific body areas. Although no study has so far provided unequivocal evidence, it is assumed that this optic signaling is used as a channel of communication between individuals that is concealed from (at least some of) their predators.
Tool usage, mimicry, and deception
While there is still no unambiguous evidence of consciousness in octopuses, their behavior is sometimes suggestive of a high level of intelligence. Like great apes and corvids, octopuses are able to use tools and can also make use of deceptive behavior. Australian researchers have also reported a type of octopus (known as the “coconut octopus”, Amphioctopus marginatus) that carries around coconut half-shells, assembling them as a shelter only when needed. In the absence of external protection these coconut shells provide the animal with a defensive tool against predators in the particularly hostile waters of the Indo-Pacific Ocean.
Researchers in Indonesia have observed what is known as the “mimic octopus”, Thaumoctopus mimicus, which displays a dramatic repertoire of postures and body patterns; several of these are clearly impersonations of venomous animals that share the same habitat. This species is able to disguise itself as a flat fish, a lion fish, or even a sea snake, which are all very aposematic animals (i.e. animals that signal the presence of dangerous toxins by conspicuous coloration). It is currently unclear whether this behavior is merely instinctive or whether some higher-level cognitive processes are also involved. In support of the latter, some defensive behavior in juvenile cuttlefish has been shown to be dependent on early experiences involving learning and memory processes.
Deceptive behavior is also exhibited by cuttlefish. Sneaker males of the giant cuttlefish Sepia apama transiently display a female body pattern leading to immediate fertilization success, even in the presence of a consort male. This sexual mimicry allows small males to reproduce despite a high rate of rejection by females of copulation attempts and despite the strong mate-guarding behavior of consort males. Even more schizophrenic are the male S. plangon cuttlefish, which display male courtship patterns to receptive females on one side of the body while simultaneously displaying female patterns to a solitary rival male on the other side, thus preventing the rival from disrupting courtship.
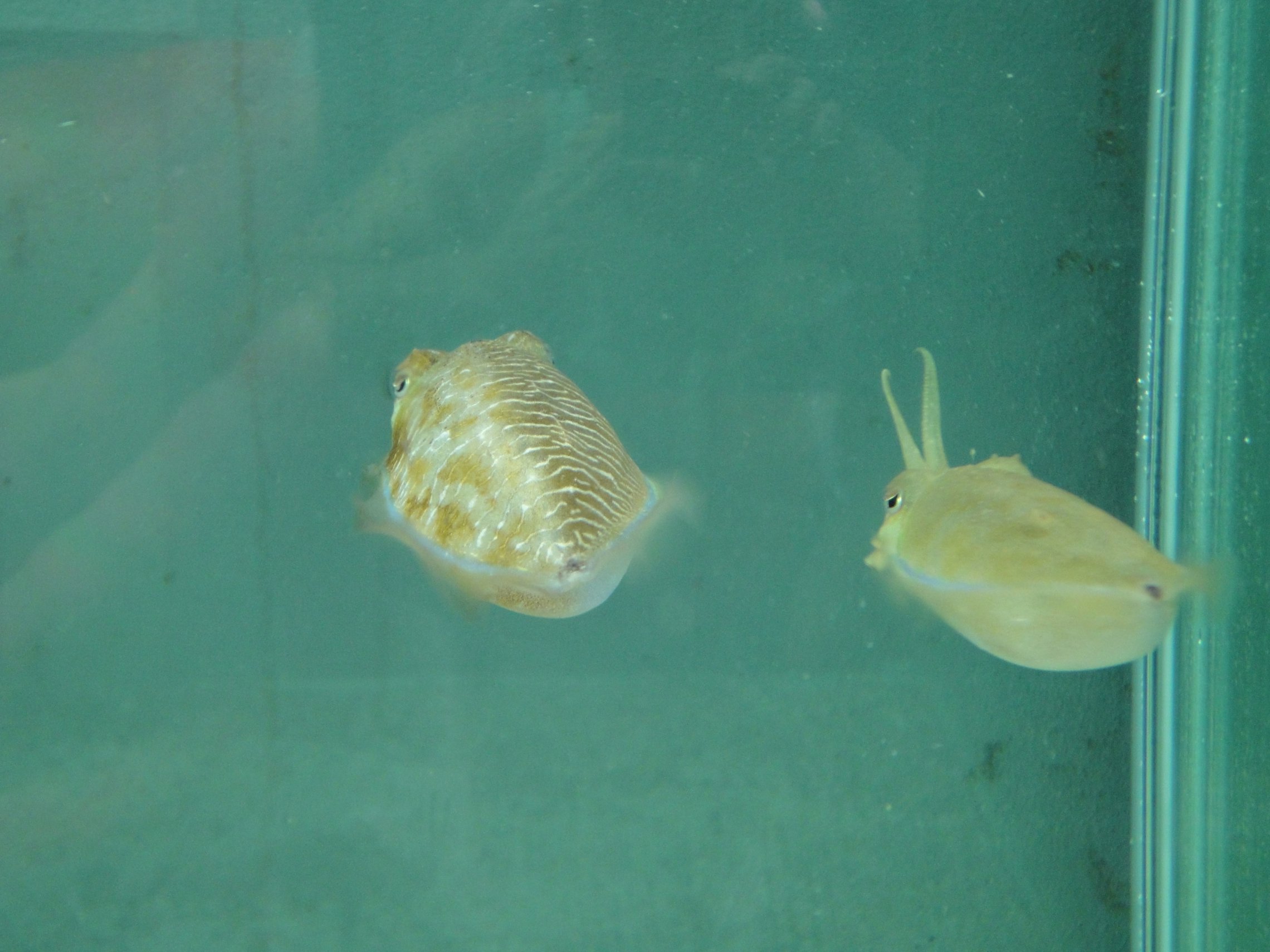
It is interesting to note that this deceptive tactic is only used when there is only one rival male present, the risk of being unmasked being too high otherwise.
Play and innovation
The constraints and challenges of a social life have often been put forward as the reason for the development of a complex brain and sophisticated cognitive abilities. However, despite the fact that most cephalopod species are solitary (setting aside gregarious squids), the experiments and observations reported above indicate that cephalopods have complex neural capacities. Another factor that can explain the emergence of complex brains is innovation. In primates and birds, the relative size of the brain has been positively correlated with innovation rates. In 2012 a walker at Victoria’s Ogden Point breakwater (Canada) photographed a giant octopus about 1 m in diameter attacking a sea gull that was on the water's surface, and drawing it underwater within a minute. This behavior is considered to be innovative since it is rather unusual for octopuses to prey on terrestrial or air-borne animals.
Finally, not only mammals (or vertebrates in general) can play: octopuses also can! They spontaneously explore new objects with their suckers and arms, and sometimes they can be observed manipulating objects from one arm to another or playing with air bubbles coming out of an aquarium air stone.
Early learning
One characteristic of cephalopods is the lack of direct parental care for juveniles (although some female octopuses take care of their eggs) and the absence of overlapping generations because parents die before their offspring hatch. A question that then arises is, how do juveniles cope with environmental constraints and challenges? Depending on the species, the egg capsules can be stained black by the female’s ink (for example with Sepia officinalis); it has been noted that in such cases the eggs have been laid in open water. In other species (e.g. Sepia pharaonis) the egg capsules are translucent and those eggs are laid under rocks or rock-shelves, out of the reach of predators; the effect of this difference in egg coloration is currently being investigated.
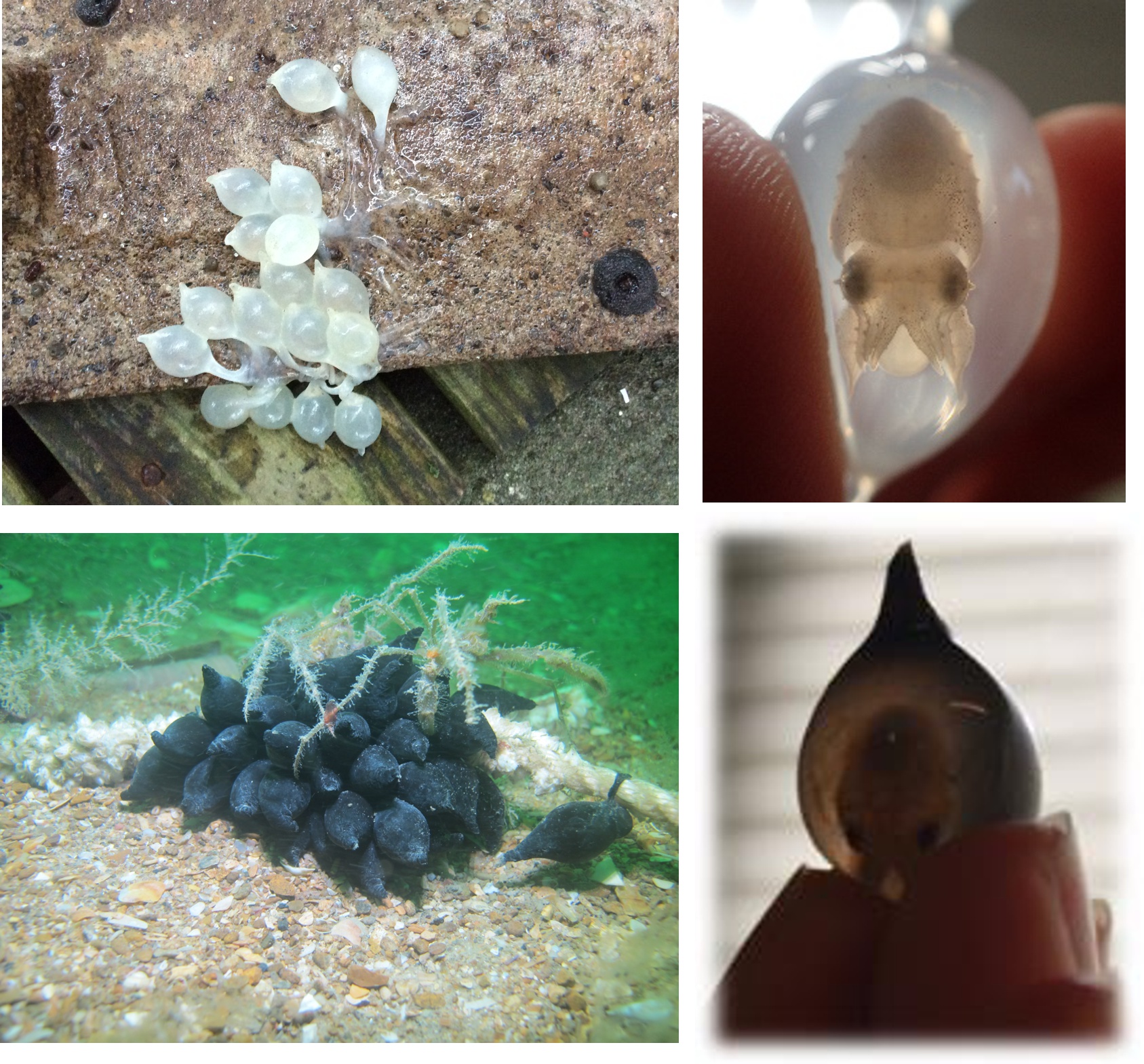
In S. officinalis it has been shown that the developmental order of at least three of the five sensory systems follows the same sequence as that of birds and mammals, with the visual system being the last to mature. In addition, both direct evidence (observation of the embryo) and indirect evidence (postembryonic effects of embryonic experience) indicate that cuttlefish embryos perceive and respond to external chemical and visual stimuli. They can learn to recognize a potential threat while they are still inside the egg; they can also learn to recognize suitable prey before they hatch and and then start feeding preferentially on this type of prey after hatching. Moreover, embryos and juveniles can distinguish prey on the basis of shape or contrast, for example by categorizing “crab” versus “shrimp” or “dark crab” versus “light crab”. Cuttlefish and octopuses are able to learn not to attack an unreachable or distasteful prey, and to discriminate between different objects through recognition of tactile cues. Information acquired during these learning processes is long-lasting, which attests to a good long-term memory.
In summary, the brains of cephalopods and their behavior are considered by behavioral biologists to be exceptional within the animal kingdom. The sophistication of their behavioral adaptations makes them promising models in the field of comparative behavioral research. The cephalopod research community is currently investigating the mechanisms of natural selection that led to molluscs developing such large brains and higher-order neural capacities, and whether their intelligence is comparable to that of some vertebrate species or whether they have developed a unique brain architecture that is associated with specific forms of intelligence not seen in other taxa. Tackling these questions will also address the issue of whether or not cephalopods can experience negative and positive emotions, and the existence of (primordial) forms of consciousness.
Much more remains to be discovered about these amazing creatures and the more they are studied the more they are respected, to such an extent that they are now protected by law within the European Union.
Further reading on this topic:
Darmaillacq A-S, Dickel L, and Mather JA (eds.) Cephalopod cognition (2014) Cambridge University Press
Responses